Kinins form a family of small peptides which are endogenously synthesized in response to pathologic processes such as pathogen infection, tissue damage, inflammation as well as allergic reactions. In response to such insults the kallikrein-kinin system is activated, whereby kallikrein, a tissue or plasma serine protease cleaves high molecular weight kininogen to yield two low molecular weight peptides: plasma bradykinin (BK), a nine amino acid peptide and tissue kallidin (KD, Lys-BK) a decapeptide3,4. Bradykinin and Kallidin are further processed by carboxypeptidases to yield des-Arg9-BK and Lys-des-Arg9-BK respectively8. Bradykinin and Kallidin both have very short half lives and are therefore rapidly degraded by endoproteases, yielding inactive byproducts. The release of these four peptides mediating pro-inflammatory activities, are agonists for B1 and B2 receptors, belonging to G-protein coupled receptor superfamily, structurally characterized by a seven transmembrane spanning domain. Bradykinin and Kallidin are both specific ligands for B2 receptors while their end products, des-Arg9-BK and Lysdes-Arg9-BK, bind and specifically activate B1 receptors4. Both B1 and B2 receptors have been shown to couple Gq and Gi thereby leading to increases in intracellular Ca2+ concentration, activation of phospholipase C, arachidonic acid release and NO production, classical inflammatory responses3,4.
Both receptors have been cloned from many different organisms and most of the structural input specific to these receptors has been acquired from mutagenesis and chemical crosslinking3.
They are distributed across the central nervous system, as well as in peripheral tissues such as vascular cells, non vascular smooth muscle, fibroblasts, epithelial cells and also expressed in some tumors4.
B2 receptor is expressed in a constitutive manner. Its activation leads to a transient increase in the intracellular concentration of Ca2+. Activation of this receptor is quick and a is similarly desensitized via internalization1,2,5.
B1 receptor is very different from B2 at many levels. First, its expression is generally induced upon inflammatory signals. However, some regions like the spinal cord, areas of the brain as well as aortic and pulmonary artery endothelial cells (from bovine) constitutively express B1 receptor. B1 expression upregulation is intimately dependent on the transcription factor NF-κB, activated in response to inflammatory signals such as interleukin-1, TNF-α, TGF-β and IFN-γ. Indeed, in vivo and in vitro inhibition of NF-κB prevents the expression of B1receptor6,7. Second, the activation of B1 receptor leads to a much more sustained effect; the increase in intracellular Ca2+ concentration is observed for a longer period of time, and the receptor does not undergo desensitization/internalization. It seems though the activated receptor undergoes aggregation in order to amplify the signaling response1. The fact that B1 receptor activation is sustained makes it an attractive potential target in the development of antagonists as it is involved in pain sensation, inflammation and perhaps diabetes4.
Alomone Labs offers specific antibodies to both B1 and B2 receptors. Anti-B1 Bradykinin Receptor (BDKRB1) Antibody (#ABR-011) and Anti-B2 Bradykinin Receptor (BDKRB2) Antibody (#ABR-012) both enable the detection of their respective receptor using western blot and immunohistochemistry applications. Alomone Labs is also pleased to introduce Bradykinin (#GPB-200), the B2 receptor ligand.
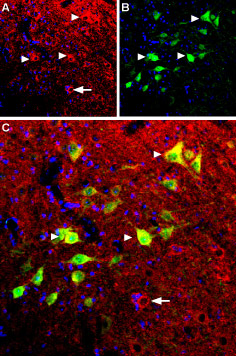
Immunohistochemical staining of mouse spinal cord using Anti-B2 Bradykinin Receptor (BDKRB2) Antibody (#ABR-012). A. B2 bradykinin receptor (red) appears in several spinal cord neurons (triangles). B. Parvalbumin (green) appears in neurons. C. Merge of B2 bradykinin receptor and parvalbumin shows co-expression in several neurons (triangles) but not all (arrow in C). DAPI is used as the counterstain (blue).
Experimental procedure and figure processed at Alomone Labs.
References
- Calixto, J.B. et al. (2004) Br. J. Pharmacol. 143, 803.
- Faussner, A. et al. (1999) Immunopharmacology 45, 13.
- Kuhr, F. et al. (2010) Neuropeptides 44, 145.
- Leeb-Lundberg, L.M. et al. (2005) Pharmacol. Rev. 57, 27.
- Marceau, F. et al. (2002) Int. Immunopharmacol. 2, 1729.
- Sardi, S.P. et al. (2000) Acta Pharmacol. Sinica 21, 105.
- Sardi, S.P. et al. (2002) J. Pharmacol. Exp. Ther. 301, 975.
- Skidgel, R.A. and Erdös, E.G. (1998) Immunol. Rev. 161, 129.