The contribution and importance of K+ channels to the normal activity of the heart is best exemplified by disturbances in the activity of one K+ channel, causing cardiac arrhythmia. This voltage dependent cardiac K+ channel is called hERG (KV11.1) and both mutations in the gene encoding this channel (KCNH2) and drugs that block the channel might cause arrhythmia and death. However, cardiomyocytes express several different K+ channels, all contributing to the electrical control of the cardiac muscle contraction-relaxation cycle. The repertoire of K+ channels in these cells is plastic and is changing under different physiological and pathological conditions. Below we review the use of Alomone Labs’ K+ channels antibodies and toxins in such aspects of cardiac research.
Heart beats result from nearly regular changes in intracellular Ca2+ in heart muscle cells (cardiomyocytes), with elevated Ca2+ causing muscle contraction. As in many other cellular processes, this transient Ca2+ elevation is initiated and maintained by depolarization of the cell membrane potential and is terminated by its repolarization back to resting values (in the form of an action potential waveform). Repolarization of the cardiomyocyte action potential is achieved by the delayed activation of potassium channels facilitating K+ efflux. The concerted activity of many different types of K+ channels (of which some 80 human genes are known to encode members of the four main families in this protein group), determines the precise timing and efficiency of cardiac repolarization1,2.
The use of specific antibodies serves as a tool to demonstrate the expression of ion channels in the heart, in different species and under diverse conditions.
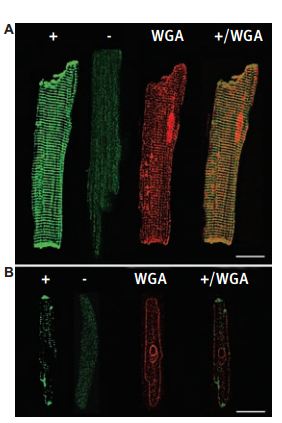
Expression of KCNK3 (TASK-1) protein in rat myocytes. Immunofluorescence images from single ventricular (A ) and atrial (B) myocytes were stained with Anti-KCNK3 (TASK-1) Antibody (#APC-024) (+), eliminated by competitive inhibition with the antigenic peptide (-), and costained with wheat germ agglutinin (WGA). Colocalization of KCNK3 and WGA is indicated in yellow (+/WGA). Bar, 15 µm.
Adapted from reference #3, with the kind permission of Dr. S.A. Jones of the University of Hull, UK and the Am. J. Physiol. Heart Circ. Physiol.
Anti-KCNK3 (TASK-1) Antibody (#APC-024) was used for demonstration (western blot and immunocytochemistry) of the expression of the channel in rat atria and ventricles (Figure 1.)3. Anti-KCNMA1 (KCa1.1) (1097-1196) Antibody (#APC-021) was used for demonstration (western blot) of the expression of the channel in mouse heart (presumably in mitochondria)4. Anti-KCNN2 (KCa2.2, SK2) Antibody (#APC-028) was used for demonstration of channel expression in mouse, cat, rat and human heart (western blot) and in mouse ventricle and atria or human atrial myocytes (immunocytochemistry)5. Anti-Kir2.1 (KCNJ2) Antibody (#APC-026) was used for demonstration (immunocytochemistry) of the expression of the channel in mouse atria and ventricles and differential subcellular localization (figure 2)6. Anti-GIRK1 (Kir3.1) Antibody (#APC-005) was used to demonstrate (western blot and immunocytochemistry) channel expression in ventricle (low expression) atria and SA node of guinea pig, ferret and rat (figure 3)7. Anti-KV3.1b (KCNC1) Antibody (#APC-014) was used to demonstrate channel expression (western blot immunocytochemistry and immunohistochemistry) mainly in dog atria and much less in ventricles8. Anti-KV11.1 (KCNH2) antibodies (#APC-016 and #APC-062) were used to demonstrate (western blot) expression in dog hearts, and the interaction (immunoprecipitation) with KCNE2 in both healthy and diseased dogs ventricles9. Anti-KCNH2 (erg1) Antibody (#APC-016) was used to demonstrate differential subcellular localization of the channel in rat atria vs ventricles (western blot in transfected cells and immunocytochemistry and immunohistochemistry form rat tissue)10. Anti-KV11.1 antibodies were used to detect (western blot) of the channel protein in human and guinea pig hearts11 and the physical interaction (western blot immunoprecipitation) of the channel with other channel proteins (KCNE1 and KV7.1) in horse atria and ventricles12. Anti-Kir2.2 (KCNJ12) Antibody (#APC-042) was used to demonstrate lack of expression (western blot) in guinea pig and sheep heart. Possibly because of low identity to the rat epitope13. Anti-M2 Muscarinic Receptor Antibody (#AMR-002) was used to demonstrate (co-immunoprecipitation) the luck of association of the receptor with Kir3.1 channels in rat atria14. In addition, Tertiapin (#STT-250) a Kir blocker, at 300 nM had no effect on basal current in mouse atrium15.
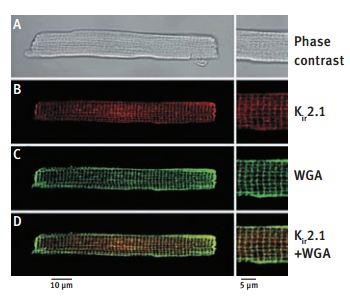
A. Phase contrast image of a mouse ventricular cell, also present in B-D, using immunofluorescence detection. B. Immunofluorescence staining of a mouse ventricular cells with Anti-Kir2.1 (KCNJ2) Antibody (#APC-026) (red). C. The green coloration corresponds to WGA staining of the cell membrane. D. Superimposition of both images shows significant colocalization (yellow) of Kir2.1 and AGA in the transverse striations, further confirming the t-tubular localization of Kir2.1.
Adapted from reference #6, with the kind permission the J. Physiol.
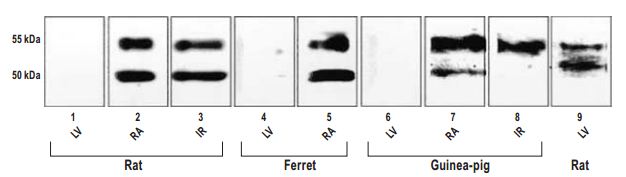
Western blot analysis of samples obtained from rat, ferret, and guinea pig heart using Anti-GIRK1 (Kir3.1) Antibody (#APC-005). One or two major bands corresponding to molecular weights of ~50 and ~55 kD were detected in RA (Lanes 2, 5, and 7) and IR (Lanes 3, 5, and 8), but not LV (Lanes 1, 4, and 6), in which equal amounts of protein from different tissues were loaded. Two similar bands were detected in LV from rat when the amount of protein was increased (Lane 9). IR, intercaval region; LV, left ventricle; RA, right atrial appendage.
Adapted from reference #7, with the kind permission the J. Histochem. Cytochem.
Many of these K+ channels are modulated by endocrine, metabolic, and neuronal factors that in turn affect cardiac parameters such as heart rate. Increasing activity of K+ channels (resulting from increased numbers of functional channels, changes in kinetics, or changes in the balance between the various channels) leads to shorter action potentials (which may facilitate faster heart rate, for example). On the other hand, reduced activity that supports K+ efflux leads to broader action potentials.
Several K+ channel genes that are expressed in cardiomyocytes harbor mutations strongly associated with cardiac diseases. Sometimes, such mutation may lead to reduced channel expression. However, pharmacological rescue is possible. The calcium ER SERCA pump blocker, Thapsigargin (#T-650) and E-4031 (#E-500) a specific KV11.1 blocker were used to rescue the expression of Long QT (interval)2 mutated KV11.1 channels16. E-4031 was also used to block background KV11.1 currents to better asses KCa2 contribution to cardiomyocyte action potential5.
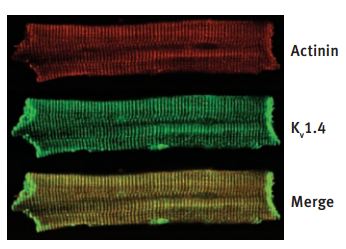
cKV1.4 protein expression in Mid layer of normal and failing left ventricles. immunocytochemical stain of a representative Mid cell with Anti-KV1.4 Antibody (#APC-007) and α-actinin demonstrates colocalization of the proteins.
Adapted from reference #25, with the kind permission of Dr. G. F. Tomaselli of Jones Hopkins University, USA and the Am. J. Physiol. Heart Circ. Physiol.
In addition, remodeling of the K+ channel repertoire in different regions of the heart may be the result or the cause of certain diseases. In some cases, correlation between cardiac condition (in human patients and/or animal models) and K+ channel remodeling was demonstrated. Many examples for such remodeling are given by the use of specific antibodies. Anti-Kir 2.1 (KCNJ2) Antibody (#APC-026) was used to show channel upregulation (western blot from different groups heart tissue) only in human patients with atrial fibrillation17. The antibody was also used to probe channel expression western blot) in wild type vs ANKB+/- mice (Long QT (interval)4)18 and in castrated vs. normal male mice,19 but in both cases no change was found. Anti-Kir2.3 (KCNJ4) Antibody (#APC-032) was used to probe channel expression (western blot) in wild type Vs ANKB+/- mice (Long QT (interval)4), but no change was found18. Anti-GIRK1 (Kir3.1) Antibody (#APC-005) was used to demonstrate (western blot) the absence of protein in Kir3.1 knockout mice20 and to demonstrate (western blot) reduced protein expression in atria from atrial fibrillation human patients21. Anti-KV1.4 Antibody (#APC-007) was used to demonstrate (western blot) protein down regulation in rabbits with long term tachycardia22 but show no change in rabbits with failing hearts compared to controls23. It was used also to demonstrate (western blot) increased protein expression in mice, which are a model for cardiac hypertrophy and heart failure24 as well as increased protein expression (western blot and immunocytochemistry) in dogs, with tachycardia-induced heart failure (Figure 4.)25. Anti-KV1.5 (KCNA5) Antibody (#APC-004) was used to demonstrate (western blot) reduced protein expression in atria from atrial fibrillation human patients21 and to demonstrate (western blot) absence of the protein in dog atria8 (but see26) Anti-KV2.1 Antibody (#APC-012) was used to demonstrate (western blot) up regulation of the channel in hearts of KV1.5 knockout mice27. It was also used to detect no change in protein level (western blot) in castrated vs. normal male mice19. Anti-KV4.2 Antibody (#APC-023) was used to detect similar protein levels (western blot) in castrated vs. normal male mice19. Anti-KV4.3 Antibody (#APC-017) was used to demonstrate (western blot) reduced protein expression in atria from atrial fibrillation human patients21 and decreased protein expression (western blot, accompanying lower K+ current, and longer QT intervals) in late pregnant mice compared to nonpregnant28. Using the antibody it was fond that there is no change in protein level (western blot) in dogs hearts after ischemia29 or in castrated vs. normal male mice19. In expression system and rat heart it was used to demonstrate co-localization of channel with auxiliary KchAP (Figure 5.)30. Anti-KV11.1 (KCNH2) antibodies were used to demonstrate increased protein expression (western blot) in dogs, with tachycardia-induced heart failure25 or in heart failure induced dog ventricular myocytes and further increase in response to TNF-α.31 Anti-KCNE1 Antibody (#APC-163) was used to probe channel expression (western blot) in wild type Vs ANKB+/- mice (Long QT (interval)4) were no change was found18. In addition it was used to demonstrate (western blot immunoprecipitation) physical interaction of the channel with other channel proteins (KV11.1 and KV7.1) in horse atria and ventricles12. Anti-KCNE2 Antibody (#APC-054) was used to demonstrate (western blot) expression in dog, rat and human hearts, the protein is down regulated in diseased hearts of dogs. It was also used to demonstrate (immunoprecipitation) interaction with KV11.1 in both healthy and diseased dogs ventricles (figure 6). The protein level is increased with age (in dog) in correlation to increased If (HCN2)9.
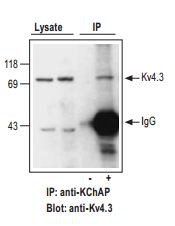
Adult rat heart lysates were incubated with (+) or without (-) KChAP antibody (1:100 dilution). Immunoprecipitates (IP) were collected on Dynabeads and presence of KV4.3 channels probed by Western blotting. Western blot with Anti-KV4.3 Antibody (#APC-017) (1:150). Lysate (40 µg protein loaded) and IP (- and + anti-KChAP) are shown.
Adapted from reference #30, with the kind permission of Am. J. Physiol. Cell Physiol.
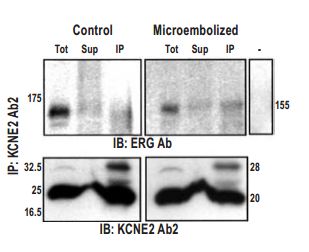
Coimmunoprecipitation of ERG with KCNE2 using Anti-KCNE2 Antibody (#APC-054) (Ab2) from membrane proteins prepared from control and microembolized canine ventricles. Lanes Tot, Sup, IP, and – represent total membrane protein, supernatant, immunoprecipitate, and immunoprecipitate in absence of Ab2, respectively.
Adapted from reference #9, with the kind permission the journal Circulation.
References
- Bers, D.M. (2000) Circ. Res. 87, 275.
- Nattel, S. et al. (2007) Physiol. Rev. 87, 425.
- Jones, S.A. et al. (2002) Am. J. Physiol. Heart Circ. Physiol. 283, H181.
- Wang, X. et al. (2004) Am. J. Physiol. Heart Circ. Physiol. 287, H2070.
- Xu, Y. et al. (2003) J. Biol. Chem. 278, 49085.
- Clark, R.B. et al. (2001) J. Physiol. 537, 979.
- Dobrzynski, H. et al. (2001) J. Histochem. Cytochem. 49, 1221.
- Yue, L. et al. (2000) J. Physiol. 527, 467.
- Jiang, M. et al. (2004) Circulation 109, 1783.
- Rasmussen, H.B. et al. (2004) Am. J. Physiol. Heart Circ. Physiol. 286, H1300.
- Zicha, S. et al. (2003) Am. J. Physiol. Heart Circ. Physiol. 285, H1641.
- Finley, M.R. et al. (2002) Am. J. Physiol. Heart Circ. Physiol. 283, H126.
- Dhamoon, A.S. et al. (2004) Circ. Res. 94, 1332.
- Nikolov, E.N. and Ivanova-Nikolova, T.T. (2004) J. Biol. Chem. 279, 23630.
- Lomax, A.E. et al. (2003) Br. J. Pharmacol. 140, 576.
- Anderson, C.L. et al. (2006) Circulation 113, 365.
- Gaborit, N. et al. (2005) Circulation 112, 471.
- Mohler, P.J. et al. (2003) Nature 421, 634.
- Brouillette, J. et al. (2003) J. Physiol. 546, 403.
- Bettahi, I. et al. (2002) J. Biol. Chem. 277, 48282.
- Brundel, B.J. et al. (2001) J. Am. Coll. Cardiol. 37, 926.
- Tsuji, Y. et al. (2006) Circulation 113, 345.
- Rose, J. et al. (2005) Am. J. Physiol. Heart Circ. Physiol. 288, H2077.
- Bodi, I. et al. (2003) J. Am. Coll. Cardiol. 41, 1611.
- Akar, F.G. et al. (2005) Am. J. Physiol. Heart Circ. Physiol. 288, H2887.
- Fedida, D. et al. (2003) Circ. Res. 93, 744.
- London, B. et al. (2001) Circ. Res. 88, 940.
- Eghbali, M. et al. (2005) Circ. Res. 96, 1208.
- Liu, X.S. et al. (2007) Am. J. Physiol. Heart Circ. Physiol. 292, H560.
- Kuryshev, Y.A. et al. (2000) Am. J. Physiol. Cell Physiol. 278, C931.
- Wang, J. et al. (2004) J. Biol. Chem. 279, 13289.