The role of NaV Channels in several types of pain opens a gateway for the development of specific sodium-channel inhibiting agents for the treatment of chronic pain. In this short piece we demonstrate the use of Alomone Labs NaV-related products in pain research. Right: DRGs play a pivotal role in nociception.
Introduction
Pain sensation is an extremely common pathophysiological condition. The voltage-gated sodium (NaV) channel isoforms NaV1.7, 1.8 and 1.9 are selectively expressed in pain-conducting pathways of the peripheral nervous system (PNS). These isoforms are mostly localized to peripheral sensory ganglia, including dorsal root ganglia (DRG) and trigeminal ganglia (TG). NaV1.7 is specifically associated with pain pathways since it is highly expressed in both unmyelinated C-fibers that contain the neuropeptide substance P and in free nerve endings in nociceptive receptor fields.
The association between NaV channels and pain is further demonstrated by individuals who carry NaV1.7 mutations. Some mutations, completely abolish nociception and these individuals do not experience pain.
NaV channels have also been implicated in inflammatory pain states. Multiple studies have shown changes in sodium channels in experimental animals with inflammatory lesions. Inflammation typically causes increased expression of the NaV1.3, 1.7, 1.8, and 1.9 isoforms in sensory neuronal cell bodies.
The role of NaV channels in several types of pain opens a gateway for the development of specific sodium-channel inhibiting agents for the treatment of chronic pain2.
NaV Channels and Pain
Resurgent Na+ currents are currents activated during repolarization, and are the driving force for the generation of additional action potentials and thus contribute to repetitive firing of neurons. TTX-sensitive (TTX-s) resurgent currents are detected in cerebellar and dorsal root ganglia (DRG) neurons, and are achieved in a NaV1.6-dependent manner . A novel TTX-resistant (TTX-r) resurgent Na+ current was isolated in rat DRGs . In many aspects this current is similar to the TTX-s resurgent current in that it requires the presence of NaVβ4 subunit. However, TTX-r resurgent currents exhibit slower kinetics and occur at more depolarized voltages. They are also sensitive to a NaV1.8 specific blocker. The involvement of NaV1.8 in resurgent currents is further strengthened by immunoprecipitation studies using Anti-NaV1.8 (SCN10A) Antibody (#ASC-016) in rat DRG lysates. NaV1.8 was shown to interact with NaVβ4. These TTX-r resurgent currents may contribute to the membrane excitability of nociceptive DRG neurons under normal conditions. The increase in both types of resurgent currents by inflammatory mediators could contribute to neuronal hyperexcitability associated with inflammatory pain8.
Pain-related bone cancer is caused in part by increased excitability of DRG neurons. Western blot analysis and electrophysiological recordings of rat DRGs show that NaV1.8 channel expression and activity are upregulated in DRG neurons, and contribute to the development of cancer-induced bone pain3. Similar data is observed for NaV1.9, for which changes in protein levels are significant, as observed in western blot analysis and immunohistochemistry using Anti-SCN11A (NaV1.9) Antibody (#ASC-017) (Figure 1)4.
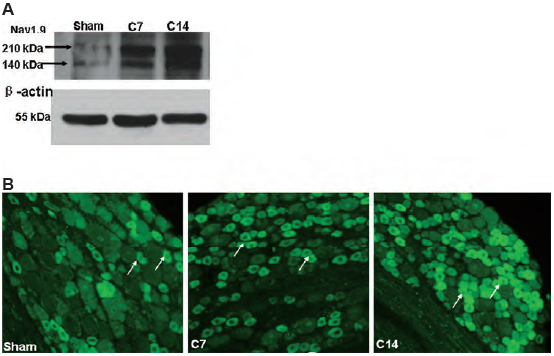
A. Western blot analysis of rat DRG lysates using Anti-SCN11A (NaV1.9) Antibody (#ASC-017).
B. Immunohistochemical staining of rat DRG sections using same antibody as in A. Both, western blot and immunostaining show that NaV1.9 expression significantly increases in DRGs 14 days following bone cancer induction (C14), compared to sham-treated rats.
Adapted from reference 4 with permission of Elsevier.
Painful diabetic neuropathy is a complication from which diabetic patients can suffer from. It is believed to originate in the peripheral system. A study showed that NaV1.7 and NaV1.8 channels are both upregulated in small DRGs and in peripheral nociceptive C-fibers in both immunohistochemistry and western blot analyses using Anti-NaV1.8 (SCN10A) Antibody and Anti-NaV1.7 (SCN9A) Antibody (#ASC-008) (Figure 2). The increased excitability in small DRGs from diabetic rats might underlie the decreased conduction in the diabetic high-firing-frequency polymodal C-fibers, thus uncovering a novel mechanism for hyperalgesia associated with diabetes7.
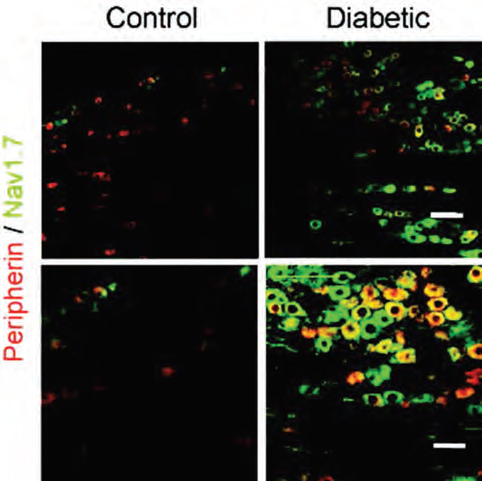
Immunohistochemical staining of rat DRG sections using Anti-NaV1.7 (SCN9A) Antibody (#ASC-008). NaV1.7 expression (green) is higher in DRGs from diabetic rats (right panels) compared to control rats (left). C-fibre marker peripherin is labeled in red. Top: x20 magnification. Bottom: x40 magnification.
Adapted from reference 7 with permission of Oxford University Press.
In a study, nociceptive sensory neurons were generated from HUES6 embryonic stem cells. Characterization of the cells was achieved in part by immunocytochemical staining of the derived nociceptors using Anti-TRPV1 (VR1) Antibody (#ACC-030) and Anti-SCN11A (NaV1.9) Antibody. These cells also express cardiac specific NaV1.5 channels, stained using Anti-NaV1.5 (SCN5A) (1978-2016) Antibody (#ASC-013), reinforcing its role during development. This work demonstrates that nociceptors can be derived from human pluripotent stem cells (hPSCs) and furthermore establishes a platform for studying developmental processes in nociceptive neurons and the possibility of developing targeted pharmacology1.
Studies show that inflammatory cytokines are elevated in neuropathic pain. Furthermore, administration of inflammatory cytokines induces neuropathic pain which is also accompanied by an increase in pain-related NaV channel expression. IL-10, an anti-inflammatory cytokine was shown to reverse the effects of inflammatory cytokines, and furthermore decrease the expression of NaV1.3, NaV1.6 and NaV1.8 channels as shown in immunocytochemistry and western blot analyses of rat DRGs using Alomone Labs Anti-SCN3A (NaV1.3) Antibody (#ASC-004), Anti-NaV1.6 (SCN8A) Antibody (#ASC-009) and Anti-NaV1.8 (SCN10A) Antibody. Results suggest that down-regulating NaV channels might contribute to the effects of IL-10 in neuropathic pain5.
KIF5, a kinesin, is responsible for mediating the plus end-directed, microtubule-dependent transport of cargo proteins. It also plays an important role in transporting ion channels across long distances in axons. Following peripheral inflammation or nerve injury, NaV1.8 accumulates in peripheral nerves. KIF5 was found to be responsible for transporting NaV1.8 to the plasma membrane and axons in dorsal root ganglion (DRG) neurons. NaV1.8 co-immunoprecipitates with KIF5 and both proteins immuno-colocalize in DRG and in the sciatic nerve as shown in immunohistochemical staining using Anti-NaV1.8 (SCN10A) Antibody (Figure 3). This study provides a mechanism for Na 1.8 accumulation following inflammation6.
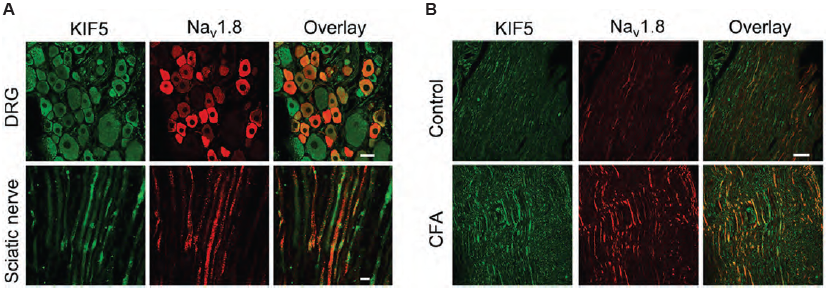
Immunohistochemical staining of rat DRG and sciatic nerve using Anti-NaV1.8 (SCN10A) Antibody (#ASC-016). A. NaV1.8 (red) and KIF5B (green) co-localize in DRG and sciatic nerve. B. NaV1.8 and KIF5B expression increases following inflammation induction.
Adapted from reference 6 with permission of the Society for Neuroscience.
References
- Eberhardt, E. et al. (2015) Stem Cell Rep. 5, 305.
- Levinson, S.R. et al. (2012) Muscle Nerve 46, 155.
- Liu, X.D. et al. (2014) PLoS ONE 9, e114623.
- Qiu, F. et al. (2012) Neurosci. Lett. 512, 61.
- Shen, K.F. et al. (2013) Exp. Neurol. 247, 466.
- Su, Y.Y. et al. (2013) J. Neurosci. 33, 17884.
- Sun, W. et al. (2012) Brain 135, 359.
- Tan, Z.Y. et al. (2014) J. Neurosci. 34, 7190.