Introduction
Many studies of ion channels expressed by neural tissues have focused on channels gated by ligands or voltage. In this mini review, another relatively new class of ion channels will be discussed – those known more for their constitutive activity than for gating properties. Prior to 1995, K+ channel subunits had been isolated with one pore domain that encoded voltage-gated or inward rectifier channels. However, that year a yeast K+ channel subunit was identified that contained two pore domains in tandem within its primary amino acid sequence7. When expressed, functional channels were formed whose activation was coupled to the equilibrium potential for K+ and passed large amounts of outward current at depolarized potentials. Accordingly, this channel was named TOK1 (two P domain outward rectifier K+ channel). TOK1 is the only K+ channel in yeast, and no tandem pore domain subunits have been identified in lower organisms.
We now know that tandem pore domain subunits are abundant in higher genomes. Of the 90 K+ channels of the 100 Mb genome of C. elegans, over 40 contain tandem pore domains. Only one of these has been cloned and functionally expressed, and has properties that resemble the mammalian channel TASK-1 (see figure 1). The 120 Mb Drosophila genome contains 9 tandem pore domain subunits. One of these subunits (ORK1) was cloned by complementation in yeast defective for K+ transport5. The tandem pore domain K+ channel KCO1 of Arabidopsis contains two EF-hand motifs and is activated by nanomolar cytosolic free calcium3.
Mammalian tandem pore channels are characterized by four putative transmembrane domains flanking two pore regions. There are several emerging structural themes within this basic membrane topology.
First, many of the pore regions contain atypical selectivity filters (GXG, where X is any amino acid other than tyrosine).
Second, a large extracellular loop (50-70 amino acids) exists between the first transmembrane domain TM1 and the first pore domain P1. The individual residues within this region are not well conserved and the size of the region is large relative to the corresponding segments of other K+ channels.
Third, the region following P1 is well conserved (the first post-pore domain). These subunits do not contain a sequence resembling an S4 domain for voltage-sensing and, in contrast to inward rectifier K+ channels of the Kir family4, they lack charged residues in the inner helix.
How large is the mammalian tandem pore domain family? To date, it includes the TWIK, TREK and TASK families.
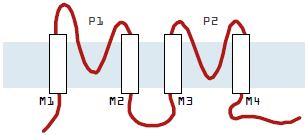
The TWIK Family
Mammalian tandem pore domain K+ subunits (KCNK: K+ channel subfamily K) have been named primarily for their functional properties. The tandem pore domain weak inward rectifier K+ channels TWIK-1 and TWIK-2 are ubiquitous2. The inward rectification of TWIK-1 is dependent on internal magnesium, similar to K+ channels of the Kir family. However, unlike the the Kir family, the amino acid residues of TWIK-1 that subtend this response have not been identified. We do know that TWIK-1 forms a covalently linked dimer in heterologous and native cells that is sensitive to reducing agents12. This structural model postulates a disulfide bridge between subunits involving extracellular cysteine residues in the TM1-P1 loop. All cloned mammalian tandem pore domain subunits except TASK-1 and TASK-3 (see below) have a conserved cysteine within the extracellular TM1-P1 loop. This suggests that, like TWIK-1, other KCNK subunits may form functional homodimeric channels via a similar disulfide bridge with four pore domains lining the ion conduction pathway.
The TREK Family
The TWIK-related K+ channel TREK-1 shares functional similarity with the Aplysia S channel, including activation by arachidonic acid and mechanical stretch19. Moreover, cAMP-mediated inhibition of TREK-1 has been observed via coupling with serotonergic receptors. The C-terminus of TREK-1 participates in a number of these fundamental properties15,16. Pharmacologic activation of TREK-1 by lipophilic molecules does not saturate at high doses, suggesting that these compounds lack a specific site of action.
A closely related channel, TREK-2, has many of the same functional properties as TREK-1 but is relatively selectively expressed in cerebellar tissue1. Both TREK-1 and TREK-2 are activated by intracellular protons, which distinguishes them from other KCNK channels. Volatile anesthetics are an important class of therapeutic agents that modulate KCNK activity (including TREK-1, TASK-1 and TASK-26,13).
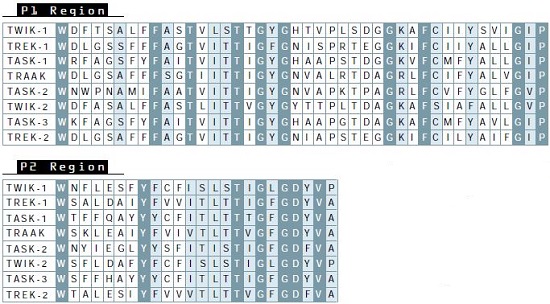
The first and second pore regions areshown as well as the first post-pore region through the end of the putative second transmembrane domain. Identical residues shared by all the channels are shown in dark gray boxes and near identical (one of two residues) in gray. Note that the second post-pore regions are not highly conserved (not shown).
The TASK Family
Extracellular protons are important modulators of a number of mammalian tandem pore domain K+ channels (TWIK-related acid sensitive K+ Channels: TASK). The TASK-1 and TASK-2 channel s are well- tuned to respond to changes in physiologic pH (apparent pK 7.3 and 7.6 external pH units, respectively), while TASK-3 channels are inhibited under more acidic conditions observed with ischemia and inflammation (pH < 7). A pore loop histidine residue has been identified which appears to function as the proton sensor of TASK-3 (this residue is conserved in TASK-1)9 ,20. While TASK-1 and TASK-3 share significant sequence homology, TASK-2 is distantly related.
TASK-1 is an open rectifier K+ channel, meaning that its currents obey the Goldman-Hodgkin-Katz current equation for an open channel11. This property is shared by TRAAK (see below). In addition to extracellular protons, intracellular protons also inhibit TASK-1 activity8. TASK-1 expression has been reported in rat brainstem and spinal cord motoneurons22. In these cells, TASK-1 activity is modulated by neurotransmitter receptors that couple to the Ga/11 class of G proteins. A functional correlate of TASK-1 is the standing outward currents (IKSO) of cerebellar granule neurons17, but the participation of TASK-3 subunits in these currents cannot yet be formally excluded.
TASK-1 may also have an important role in cardiac tissue, where it appears to generate the sustained plateau current IKp14.
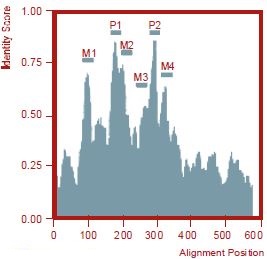
Among the transmembrane domains (M1-4), M2 is most highly conserved. A long extracellular segment precedes the first pore domain. The intracellular N-terminal and C-terminal domains are not conserved. A smooth-by-11 filter was applied after multiple sequence alignment to calculate the identity score.
TRAAK and Other KCNK Channels
TRAAK is named for its arachidonic acid sensitivity, but also is activated by a wide variety of polyunsaturated fatty acids.
Other KCNK channels also activated by polyunsaturated fatty acids include TREK-1 and TREK-21,10. TRAAK expression is restricted primarily to cell bodies of neurons, but has also been detected in axons and dendrites10,13 ,21. One structural feature that distinguishes TRAAK from other KCNK subunits is its proline-rich intracellular C-terminal domain. Both human TRAAK and KCNK7 map to chromosome band 11q13. The order and orientation of these genes has not been established at this time. However, these subunits may be under common regulatory control.
Cell-surface expression of KCNK7 has not been detected in heterologous or native cells.
Concluding Remarks
What is the role of tandem pore domain K+ channels in native cells? While tandem pore domain K+ channels are modulated by many chemical and mechanical stimuli, they are resistant to traditional inhibitors of K+ channels, most notably tetraethylammonium and 4-aminopyridine. Therefore, critical to the question of physiological role is the isolation of specific and efficacious modulators of KCNK activity.
Detailed immunolocalization studies also are needed to establish the cellular localization of tandem pore domain subunits, an area of investigation that is just now receiving attention. Finally, we anxiously await reports of mammalian gene knockout experiments (to date, we know that TOK1 is not essential for yeast growth).
References
- Bang, H. et al. (2000) J Biol Chem 275, 17412.
- Chavez, R.A. et al. (1999) J Biol Chem 274, 7887.
- Czempinski, K. et al. (1997) EMBO J. 16, 2565.
- Doupnik, C.A. et al. (1995) Curr Opin Neurobiol 5, 268.
- Goldstein, S.A. et al. (1996) Proc Natl AcadSci USA 93, 13256.
- Gray, A.T. et al. (2000) Anesthesiology 92, 1722.
- Ketchum, K.A. et al. (1995) Nature 376, 690.
- Kim, Y. et al. (1999) Am J Physiol 277 (5 Pt 2), H1669.
- Kim, Y. et al. (2000) J Biol Chem 275, 9340.
- Lauritzen, I. et al. (2000) EMBO J. 19, 1784.
- Leonoudakis, D. et al. (1998) J. Neurosci 18, 868.
- Lesage, F. et al. (1996) EMBO J. 15, 6400.
- Lesage, F. et al. (2000) FEBS Letters 471, 137.
- Lopes, C.M.B. et al. (2000) J Biol Chem 275, 16969.
- Maingret, F. et al. (1999) J Biol Chem 274, 26691.
- Maingret, F. et al. (2000) J Biol Chem 275, 10128.
- Millar, J.A. et al. (2000) Proc Natl Acad Sci USA 97, 3614.
- Patel, A.J. et al. (1999) Nat Neurosci 2, 422.
- Patel, A.J. et al. (1998) EMBO J. 17, 4283.
- Rajan, S. et al. (2000) J Biol Chem 275, 16650.
- Reyes, R. et al. (2000) Neuroscience 95, 893.
- Talley, E.M. et al. (2000) Neuron 25, 399.