The transient receptor potential (TRP) superfamily, one of the largest ion channel families, consists of a diverse group of proteins. The mammalian TRP superfamily comprises six subfamilies known as TRPC (canonical), TRPV (vanilloid), TRPM (melastatin), TRPML (mucolipins), TRPP (polycystin), and the TRPA (ANKTM1) ion channels. In mammals, approximately 28 genes encode the TRP ion channel subunits.1-4 Due to their important roles in Ca2+ homeostasis, pain, and tumorigenesis, among others, TRP channels have lately been subjects of intensive research. Alomone Labs has developed antibodies against unique epitopes, intracellular as well as extracellular, of the TRP channels. The following article highlights the role that Alomone Labs antibodies play in the TRP research field.
The TRP Superfamily
TRP channels have six putative transmembranespanning domains (TM) with a pore domain between the fifth and the sixth TM. Both the N- and the C-termini of TRPs are intracellular. All assemble as tetramers.3 TRP channels are widespread, and are either specifically or ubiquitously expressed in excitable and nonexcitable cells.1-7 The selectivity of TRP channels varies widely between the different members of the family and with regard to different cations.5 TRP channels show diverse biophysical properties and gating mechanisms and play important roles in sensory physiology, being involved in almost every sensory signal initiation from pain sensation to the five senses. They are also major players in transepithelial Ca2+ and Mg2+ transport.
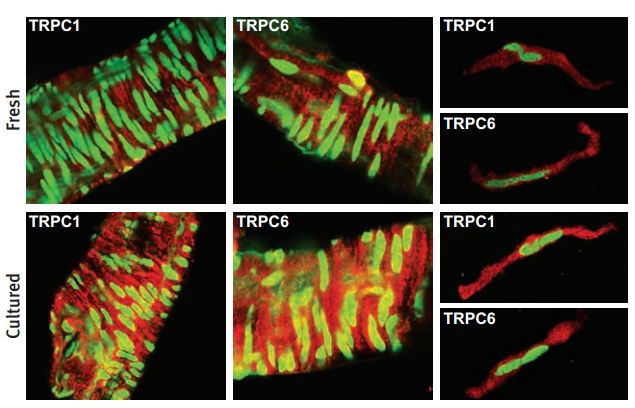
Immunohistochemical and immunocytochemical staining of rat cerebral arteries using Anti-TRPC1 Antibody (#ACC-010) and Anti-TRPC6 Antibody (#ACC-017). Immunofluorescent images showing TRPC1 staining (red) of the smooth muscle layer in whole mounts (left) and in single smooth muscle cells (right) from freshly dissected and cultured cerebral arteries. Stained smooth muscle cells are perpendicular to the longitudinal vessel direction. Note that single cells were isolated after the vessel had been cultured. Cells were costained with YOYO-1 (green).
Adapted from reference #16, with the kind permission of Dr. Hellstrand, P. of the division of Molecular and Cellular Physiology, Department of Physiological Sciences, Lund University, Lund, and the Am. J. Physiol. Cell Physiol.
The TRPC Subfamily
The TRPC subfamily consists of seven proteins named TRPC1 to 7 which can be further divided into four subgroups based on their sequence homology and functional similarities: (1) TRPC1 (2) TRPC4 and TRPC5 (3) TRPC3, TRPC6 and TRPC7 (4) TRPC2.2,8 They are highly expressed in the central nervous system and to a lesser extent in peripheral tissues. TRPC1 was the first mammalian TRP protein that was reported to form an ion channel.2,9 It can co-assemble with other TRPC subunits (TRPC3, TRPC4, TRPC5) to form heterotetramers whose properties are distinct from that of their homomeric form. The existence of the TRPC1 homomers has not been established as yet.1-3 TRPC6 can form heterotetramers with TRPC3 and TRPC7. It is primarily expressed in brain, lung and muscle. High levels of expression of the channel were also found in human platelets. Recently it was reported that TRPC6 is also expressed in the kidney where a mutated channel has been implicated in kidney failure disease.10,11 The function and regulation of the TRPC ion channels remain elusive due to conflicting data and interpretations made by independent investigators.
Alomone’s antibodies directed against the TRPC channels have been widely used for different applications, assessing expression and function of the TRPC channels.
Expression of TRPC channels in brain, peripheral tissues and cell lines was demonstrated by different methods; western blotting, immunohistochemistry and immunocytochemistry using Anti-TRPC1 Antibody (#ACC-010), Anti-TRPC3 Antibody (#ACC-016), Anti-TRPC4 Antibody (#ACC-018) and Anti-TRPC6 Antibody (#ACC-017).12-15 Expression of TRPC1 was also demonstrated by immunocytochemistry as well as western blotting in endothelium and smooth muscle of rat cerebral arteries.16
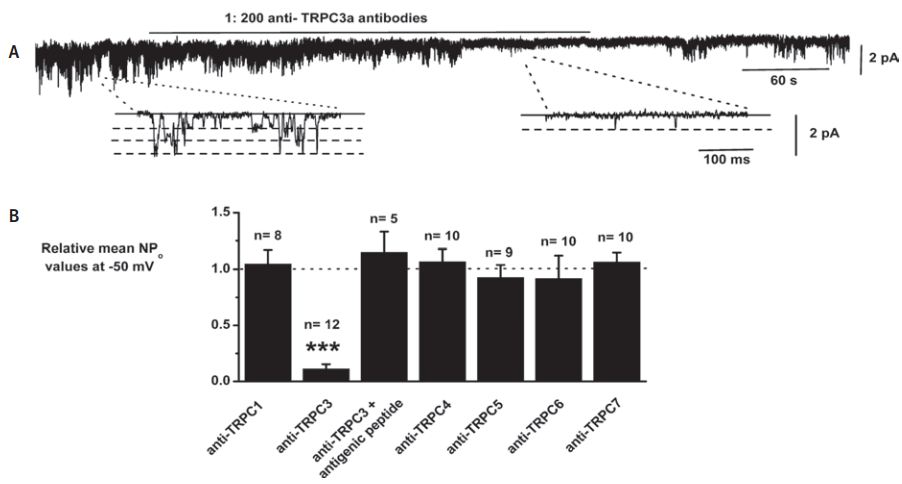
A. Bath application of, Anti-TRPC3 Antibody (#ACC-016) at 1:200 dilution reversibly inhibited constitutively active channel activity in patch held at −50 mV. Insets show individual channel currents on a faster time scale. B, mean data showing the effect of anti-TRPC antibodies: Anti-TRPC1 Antibody (#ACC-010), Anti-TRPC3 Antibody (#ACC-016), Anti-TRPC4 Antibody (#ACC-018) Anti-TRPC5 Antibody (#ACC-020) and Anti-TRPC6 Antibody(#ACC-017) on constitutive channel activity represented as NPo. The data using anti-TRPC antibodies from different sources have been pooled; note that the anti-TRPC3a antibody had no effect on channel activity following preincubation with its antigenic peptide (***P < 0.001).
Adapted from reference #14 with the kind permission of Dr. Albert, A.P. of the Ion Channels and Cell Signalling Research Centre, Division of Basic Medical Sciences, St George’s, University of London, Cranmer Terrace, London SW17 ORE, UK, and the J. Physiol.
TRPC channels have been proposed to be involved in “Store Operated Calcium Entry” (SOCE). It was demonstrated that knockdown of TRPC3 by siRNA reduced SOCE by 64%. Knocking down TRPC3 expression was assessed by western blotting using Anti-TRPC3 Antibody (#ACC-016).17 Anti-TRPC3 Antibody was demonstrated to be functional by inhibiting constitutively active channel activity in inside-out pathes.14 Reduction of Calcium entry in HUVECs was demonstrated using Anti-TRPC1 Antibody (#ACC-010).18
Recently, the proteins comprising the Store Operated Calcium complex, the Orais (Orai1 and Orai2) and the STIMs (STIM1 and STIM2) were discovered.19 However TRPC1 is still considered to be part of this complex. Co-immunoprecipitation following western blotting using the Anti-Orai1 Antibody (#ACC-060) indicated that TRPC1, Orai1, and STIM1 concertedly generate SOC channels.20
TRPC4 was shown to be necessary for neurite outgrowth in dorsal root ganglion. Its expression was increased after nerve injury and it was suggested to have an important role in sensory axonal regeneration.21 Immunohistochemical staining using the Anti-TRPC4 Antibody have demonstrated the presence of TRPC4 in adult DRG neurons.21
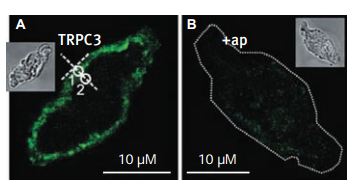
Immunocytochemical staining of TRPC3 channel proteins in rabbit ear artery myocytes using Anti-TRPC3 Antibody (#ACC-016). A. A single confocal plane fluorescence image of a myocyte labelled with anti-TRPC3a antibodies (1:200). B. Another myocyte labelled with anti-TRPC3a antibodies (1:200) preincubated with their antigenic peptide (1:100). Insets in A and B show transmitted light image of these myocytes. White circles in A indicate Regions 1 and 2, which were used to analyse the localization of fluorescence. A dotted line was used in B to outline the contour of a cell, due to its low fluorescence.
Adapted from reference #14 with the kind permission of Dr. Albert, A.P. of the Ion Channels and Cell Signalling Research Centre, Division of Basic Medical Sciences, St George’s, University of London, Cranmer Terrace, London SW17 ORE, UK, and the J. Physiol.
The TRPV Subfamily
The TRPV subfamily is comprised of six members TRPV1-TRPV6. TRPV1-TRPV4 are thermosensitive ion channels while TRPV5 and TRPV6 function as epithelial Ca2+ channels. Each of the thermosensitive ion channels exhibits distinct thermal activation thresholds, ranging from noxious cold (<17°C) to noxious heat (>52°C).22
The most established member of this family is the TRPV1 (previously also known as the capsaicin receptor or vanilloid receptor, VR1). Its involvement in thermal nociception has been well documented by different methods.23 TRPV1 is expressed predominantly in nociceptors and in sensory neurons and is activated by moderate heat (≥43°C) and by capsaicine.22-24
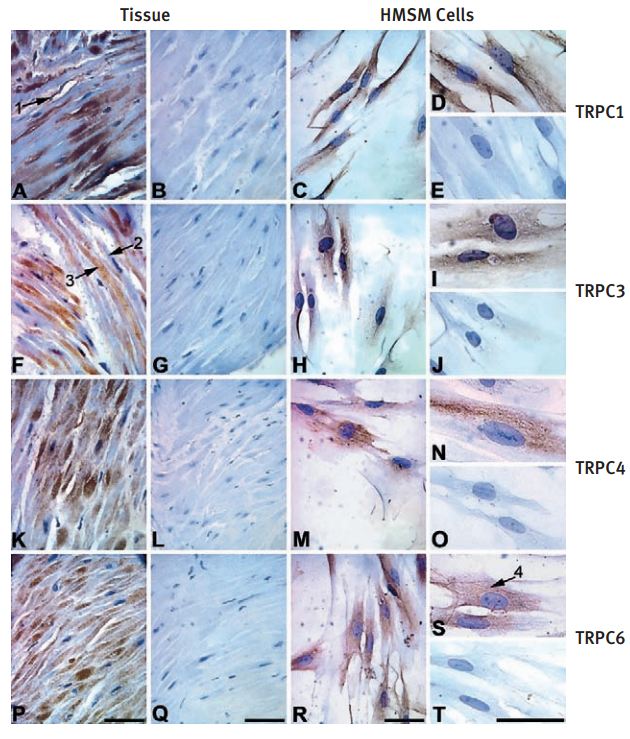
Immunohistochemical and immunocytochemical staining of term pregnant myometrial tissue and HMSM cells using Anti-TRPC3 Antibody (#ACC-016), Anti-TRPC4 Antibody (#ACC-018) and Anti-TRPC6 Antibody (#ACC-017). Intense immunostaining of TRPC1, TRPC3, TRPC4 and TRPC6 was observed in human myometrial tissue sections (A, TRPC1; F, TRPC3; K, TRPC4; P, TRPC6) and primary cultured HMSM cells (C and D, TRPC1; H and I, TRPC3; M and N, TRPC4; R and S, TRPC6). No immunostaining was observed when human myometrial tissue sections and HMSM cells were incubated with pre-immune serum (B and E, TRPC1) or pre-absorbed primary antibodies (G and J, TRPC3; L and O, TRPC4; Q and T, TRPC6).Arrow 1 = TRPC1 immunostaining in vascular tissue. Arrow 2 = limited immunostaining when nucleus is visible. Arrow 3 = intense immunostaining when the nucleus was not in the plane of focus. Arrow 4 = reticular staining in HMSM cells. Scale bars _ 50 µm.
Adapted from reference #15, with the kind permission of Dr. Tribe, R of the Parturition Research Group, Maternal and Fetal Research Unit, Department of Women’s Health, 10th Floor North Wing, Guy’s, King’s and St Thomas’ School of Medicine, St Thomas’ Hospital Campus, Lambeth Palace Road, London SE1 7EH, and the Mol. Hum. Reprod.
Expression of TRPV1 in primary culture of trigeminal ganglion and in stably TRPV1- expressing neuroblastoma SH-SY5Y cells was demonstrated both by western blotting and by immunocytochemical staining using Anti-TRPV1 (VR1) Antibody (#ACC-030).25,26
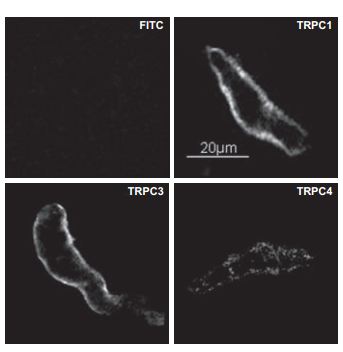
Immunocytochemical staining of TRPC1 and TRPC3 in isolated guinea pig gallbladder smooth muscle (GBSM) layer using Anti-TRPC1 Antibody (#ACC-010) and Anti-TRPC3 Antibody (#ACC-016). No immunofluorescence was evident when primary antibodies were omitted and only FITC-labeled secondary antibody was used.
Adapted from reference #13, with the kind permission of Dr. Pozo, M.J. of the department of Physiology, University of Extremadura, Ca´ceres, with the kind permission of Am. J. Physiol. Cell. Physiol.
The TRPV2 (VRL-1) however, has a higher threshold for activation by heat (≥52°C). It shares a 50% homology with TRPV1 but is not activated by capsaicin or by low pH.27
Both TRPV3 and TRPV4 are activated at a lower temperature threshold above 31°C and 25°C respectively. Both channels are expressed in DRG. However, TRPV3 is also expressed in mouse keratinocytes but not in mouse DRG, while TRPV4 is expressed in a wide variety of tissues and may also have a possible role as mechanosensitive channel.28,29 TRPV4 (also named OTRPC4) is activated under hypotonic conditions and serves as an osmo receptor. TRPV4 is expressed in brain, liver, kidney, heart, testis, dorsal root ganglion (DRG) and lung.6 Expression of the TRPV4 was demonstrated by the use of the Anti-TRPV4 Antibody (#ACC-034) in Western blotting, immunohistochemical and immunocytochemical staining.30-32
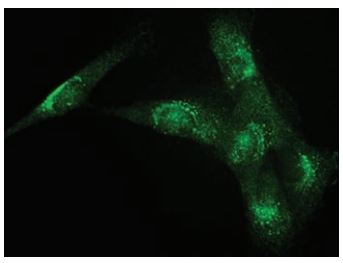
Immunocytochemical staining of human clonal SW982 synoviocytes using Anti-TRPV4 Antibody (#ACC-034) (1:400). Staining was followed by Donkey anti-Rabbit FITC (1:200).
The figure was reproduced with the kind permission of Ms. Abshire, S. and Dr. High, K. of the University of Kentucky Physiology, Lexington, KY, USA.
TRPV5 and TRPV6 form constitutively open channels, which are highly Ca2+ selective.33,34 TRPV5 is preferentially expressed in the kidney whereas TRPV6 is more prominently expressed in the intestine. TRPV6 is also expressed in human keratinocytes where it was shown to be necessary for keratinocyte differentiation. Its expression was up-regulated, both at the mRNA and protein levels following differentiation induced by 1,25-dihydroxyvitamin D3. This up-regulation in expression was demonstrated using Anti-TRPV6 Antibody (#ACC-036) in Western blotting and immunohistochemical staining.35
Although it is not expressed in benign prostate, TRPV6 was found to be upregulated in prostate cancer and correlation between expression and tumor grade was shown.36,37 In the prostate cancer cell line, LNCaP, direct involvement of TRPV6 in cell proliferation was demonstrated. Silencing the TRPV6 gene lead to a decrease in the rate of LNCaP proliferation. Silencing of TRPV6 was assessed by the use of the Anti-TRPV6 antibody.38 Both channels, TRPV5 and TRPV6 are also expressed in placenta, pancreas, salivary gland and colon.39,40
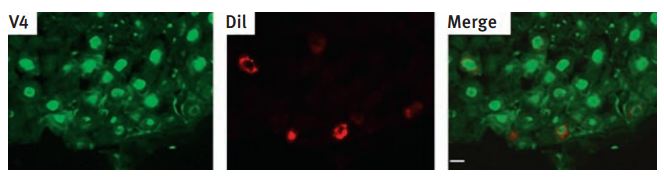
Immunohistochemical staining of TRPV4 in rat vagal pulmonary sensory neurons using Anti-TRPV4 Antibody (#ACC-034). The sections of nodose ganglion (8 µm) were fixed and subject to immunohistochemistry for the TRPV4 channel. Left: immunoreactivity for TRPV4; middle: pulmonary sensory neurons as identified by DiI labeling; right: merge of the staining for TRPV4 and DiI.
Adapted from reference #55 with the kind permission of Am. J. Physiol. Regul. Integr. Comp. Physiol.
The TRPM Subfamily
The TRPM family consist of eight members designated as TRPM1-8 that can be further divided into four pairs: TRPM1 and TRPM3; TRPM2 and TRPM8; TRPM4 and TRPM5; and TRPM6 and TRPM7.41 The family was named after the first member to be discovered, melastatin (TRPM1) whose gene was identified in metastatic and benign melanomas. TRPM channels have been suggested to play roles in tumorigenesis, proliferation, and differentiation. Recently, TRPM1 was found to be down-regulated in a highly metastatic line. The loss of TRPM1 expression has been reported to be correlated to melanoma aggressiveness.
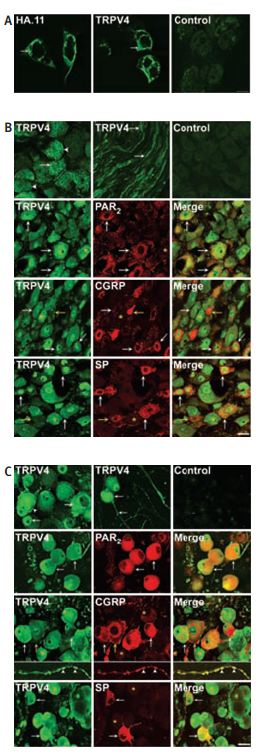
Immunocytochemical and immunohistochemical staining of TRPV4 in HEK cells and DRG neurons using Anti-TRPV4 Antibody(#ACC-034). A, localization of TRPV4a transiently expressed in HEK cells by immunofluorescence using antibodies to HA.11epitope or TRPV4. Both antibodies detected immunoreactive TRPV4. Control shows preabsorption of TRPV4 antibody with antigen used for immunization. B, localization of immunoreactive TRPV4, PAR2, CGRP or SP in sections of rat DRG. TRPV4 was detected in the soma at the plasma membrane and in intracellular locations, and also in fibres. C, Localization of immunoreactive TRPV4, PAR2, CGRP or SP in rat DRG after 2 days in culture (for a detailed legend please refer to ref #30).
Adapted from reference #30, with the kind permission of J. Physiol.
TRPM7 and TRPM6 are involved in Mg2+ homeostasis and are unique among the TRP family members, possessing a functional kinase domain at their C-terminus.6,7 Although the kinase is not necessary for the function of the channel it may have a role in modulating the activation of the channel.7 Recent work demonstrated that TRPM7 is a critical mediator of anoxic cell death.42,43
The TRPM8 is the cold (~28°C) and menthol receptor.2 TRPM8 is expressed in dorsal root ganglia (DRGs) where about 5%-10% of the small diameter DRG neurons express the channel.44,45 In DRGs, TRPM8 expressing neurons do not express the TRPV1 channel.46
Overexpression of TRPM8 was found in prostate cancer cells. However, the physiological and pathological roles that these cells play is still elusive.40 In prostate, it was suggested that TRPM8 might play a possible role in the progression of cancer to the metastatic stage.47
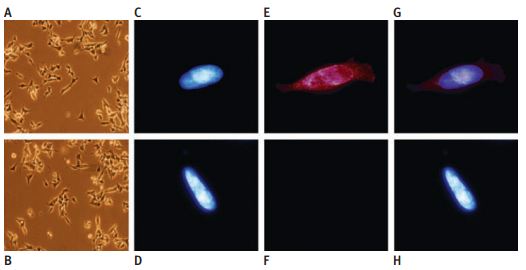
Immunocytochemical staining of TRPV1 protein expression in native and stably TRPV1-expressing SH-SY5Y neuroblastoma cells using Anti-TRPV1 (VR1) Antibody (#ACC-030). Phase contrast picture of TRPV1 cells (a) and native cells (b) showing identical morphology, 3200 magnification. Hoechst stained nucleus in TRPV1 cells (c) and native cells (d), immunization with primary TRPV1 antibodies and secondary goat anti-rabbit IgG antibodies conjugated to Alexa fluor red 568 in TRPV1 cells (e), and native cells (f) showing the TRPV1 expression in the cloned cells. (g and h) Superimposed images of c+ e and d + f, respectively.
Adapted from reference #25, with the kind permission of Dr. Lilja, J. of the department of Neurochemistry, Stockholm University, SE-106 91 Stockholm, Sweden and the Toxicol. Sci.
The TRPA Subfamily
The TRPA subfamily contains only one mammalian member, TRPA1.
TRPA1 is expressed in hair cells and dorsal root ganglia (DRG). It was originally proposed to sense painfully cold temperatures, but a more conservative assessment is that it is sensitive to membrane/cytoskeletal perturbations by cold, plant compounds such as mustard oils and perhaps stretch.48-53 Recent study describes TRPA1 receptors on nerve fibres, urothelium, and interstitial cells. Co-localization of TRPA1 using Anti-TRPA1 (extracellular) Antibody (#ACC-037) with TRPV1 and CGRP-positive nerve fibres in the urothelium along with functional studies suggest a role for TRPA1 in afferent signals from the human outflow region and involvement of TRPA1 in efferent functions of the human urethra.54
Due to their important roles in Ca2+ homeostasis, pain, and tumorigenesis, among others, TRP channels have lately been subjects of intensive research. Alomone Labs has developed antibodies against unique epitopes, intracellular as well as extracellular, of the TRP channels. Antibodies from Alomone Labs have been widely cited in the literature for a variety of applications.
References
- Moran, M.M. et al. (2004) Curr. Opin. Neurobiol. 14, 362.
- Clapham, D.E. et al. (2003) Pharmacol. Rev. 55, 591.
- Clapham, D.E. (2003) Nature 426, 517.
- Padinjat, R. and Andrews, S. (2004) J. Cell Sci. 117, 5707.
- Huang, C.L. (2004) J. Am. Soc. Nephrol. 15, 1690.
- Voets, T. and Nilius, B. (2003) J. Membr. Biol. 192, 1.
- Minke, B. and Cook, B. (2002) Physiol. Rev. 82, 429.
- Montell, C. (2001) Sci. STKE. 2001, re1.
- Zitt, C. et al. (1996) Neuron 16, 1189.
- Winn. M.P. et al. (2005) Science 308, 1801.
- Reiser, J. et al. (2005) Nat. Genet. 37, 739.
- Wang, J. et al. (2004) Am. J. Physiol. Lung Mol. Physiol. 286, L848.
- Morales, S. et al. (2007) Am. J. Physiol. Cell. Physiol. 292, C553.
- Albert, A.P. et al. (2006) J. Physiol. 571.2, 361.
- Dalrymple, A. et al. (2002) Mol. Hum. Reprod. 8, 946.
- Bergdahl, A. et al. (2005) Am. J. Physiol. Cell Physiol. 288, C872.
- Wu, X. et al. (2004) J. Biol. Chem 279, 43392.
- Ahmmed, G.U. et al. (2004) J. Biol. Chem. 279, 20941.
- Yeromin, A.V. et al. (2006) Nature 443, 226.
- Cheng, K.T. et al. (2008) J. Biol. Chem. 283, 12935.
- Wu, D. et al. (2008) J. Biol. Chem 283, 416.
- Tominaga, M. and Caterina, M.J. (2004) J. Neurobiol. 61, 3.
- Tominaga, M. et al. (2001) Proc. Natl. Acad. Sci. U.S.A. 98, 6951.
- Sugiura, T. et al. (2002) J. Neurophysiol. 88, 544.
- Lilja, J. et al. (2007) Toxicol. Sci. 99, 174.
- Fabbretti, E. et al. (2006) J. Neurosci. 26, 6163.
- Muraki, K. et al. (2003) Circ. Res. 93, 829.
- Corey, D.P. (2003) Neuron 39, 585.
- Güler, A.D. et al. (2002) J. Neurosci. 22, 6408.
- Grant, A.D. et al. (2007) J. Physiol. 578, 715.
- Yang, X.R. et al. (2006) Am. J. Physiol. Lung Cell Mol. Physiol. 290, L1267.
- Alvarez, D.F. et al. (2006) Cir. Res. 99, 988.
- Bodding, M. and Flockerzi, V. (2004) J. Biol.Chem. 279, 36546.
- Peng, J. B. et al. (2003) J. Physiol. 551, 729.
- Lehen’kyi, V. et al. (2007) J. Biol. Chem. 282, 22582.
- Fixemer, T. et al. (2003) Oncogene 22, 7858.
- Wissenbach, U. et al. (2001) J. Biol. Chem. 276, 19461.
- Lehen’kyi, V. et al. (2007) Oncogene 26, 7380.
- Hoenderop, J.G.J. et al. (2005) Physiol. Rev. 85, 373.
- Van de Graaf. S.F.J. et al. (2004) J. Steroid. Biochem. Mol. Biol. 89-90, 303.
- Fleig, A. and Penner, R. (2004) Trends Pharmacol. Sci. 25, 633.
- Schmitz, C. et al. (2005) J. Biol. Chem. 280, 37763.
- Aarts, M.M. and Tymianski, M. (2005) Neuroscientist 11, 116.
- McKemy, D.D. (2005) Molecular Pain 1, 16.
- Peier, A.M. et al. (2002) Cell 108, 705.
- Dragoni, I. et al. (2006) J. Biol. Chem. 281, 37353.
- Zhang, L. and Barritt, G.J. (2006) Endocr. Relat. Cancer 13, 27.
- Hill, K. and Schaefer, M. (2007) J. Biol. Chem. 282, 7145.
- Story, G.M. et al. (2003) Cell 112, 819.
- Bandell, M. et al. (2004) Neuron 41, 849.
- Jordt, S.E. et al. (2004) Nature 427, 260.
- Zurborg, S. et al. (2007) Nat. Neurosci, 10, 277.
- Corey, D. P. et al. (2004) Nature 432, 723.
- Gratzke C. et al. (2008) Eur. Urol. doi:10.1016/j. eururo.2008.04.042
- Ni, D. et al. (2006) Am. J. Physiol. Regul. Integr. Comp. Physiol. 291, R541.