Extracellular vesicles (EVs) have gone by many names – exosomes, micro-vesicles, cellular debris, “platelet dust” – but regardless of what we call, it’s now clear they’re actually major components of intercellular communication, with significant influence over multiple physiological processes. These naturally occurring nanoparticles, typically in the range of 50–150 nm, are released from cells where they transport lipids, proteins, nucleic acids and other molecules to other cells. EVs also appear in diseases like cancer and neurodegenerative disorders, where they can modify the disease environments, promote metastasis, or even spread pathogenic proteins.
Here we’ll take a look at how EVs research has evolved, how these tiny structures function, and consider their potential as drug delivery systems in the clinic.
A Brief History of Extracellular Vesicles
To get more of an understanding of EVs we have to go back to the 1960s with an observation of what was then referred to as “platelet dust” in blood samples (1). Initially considered cellular debris, these tiny particles slowly gathered more interest through the 1970 when researchers began to recognize their potential significance as cargo carriers in cellular processes (2). By the 1980s, the term “extracellular vesicle” emerged to encompass a range of vesicles like exosomes, micro-vesicles, and apoptotic bodies (3–5). And from there the field bloomed: the 90s brought proof that EVs are biological functional (6); by 2008 it was clear they could also transfer RNA cargo to other cells (7–9); and now we have an exciting field dedicated to the role of EVs in human health and disease.
Advances in technology were central to driving EVs research forward. Differential ultracentrifugation became and remains a key technique for isolating EVs from cell cultures and bodily fluids. Alongside isolation, new microscopy and flow cytometry techniques in the late 20th and early 21st centuries significantly improved our ability to properly see and characterize EVs. Additionally, with nanoparticle tracking analysis and other high-resolution techniques it became possible to precisely quantify and follow the behavior of EVs (10). These technologies not only showed us the intricacies of EVs biogenesis, composition, and function, but also delivered a more comprehensive and accurate understanding of their roles in cellular communication (11).
Extracellular Vesicles in Action
We now know that EVs are so much more than platelet dust or cellular debris. We have learnt that EVs make up a sophisticated cargo delivery or transport system, moving proteins, lipids, nucleic acids and other important molecules to where they modulate diverse physiological functions. For instance, EVs can transport apoptotic signals or they might carry autophagic proteins and RNAs that promote the degradation and recycling of cellular components (12, 13).
EVs were demonstrated to be involved in modulating our body’s immune response by transporting antigens, cytokines and other immune regulatory molecules (14). On the other hand, EVs are exploited in pathological processes and were found to deliver oncoproteins, pro-metastatic factors, viral and bacterial components, and other immune invasion molecules (15, 16).
EVs are also involved at more fundamental levels like protein expression (17), multiple signaling pathways, deciding cell fate (18), and even embryonic processes like epithelial-mesenchymal transition (19).
From a practical standpoint, being able to identify EVs is paramount. That’s why Alomone provides antibodies for both positive markers of EVs: Anti-CD9 (extracellular) (#ANR-209), Anti-CD63 (extracellular) (#ANR-210), and Anti-CD81 (extracellular) (#ANR-211), and for the EVs negative marker: Anti-Calnexin (#ACS-009); all of which can be conjugated directly to fluorophores. Alomone’s products are always validated; please refer to Figure 1 that shows flow cytometry results for the positive markers of EVs using our extracellular antibodies.
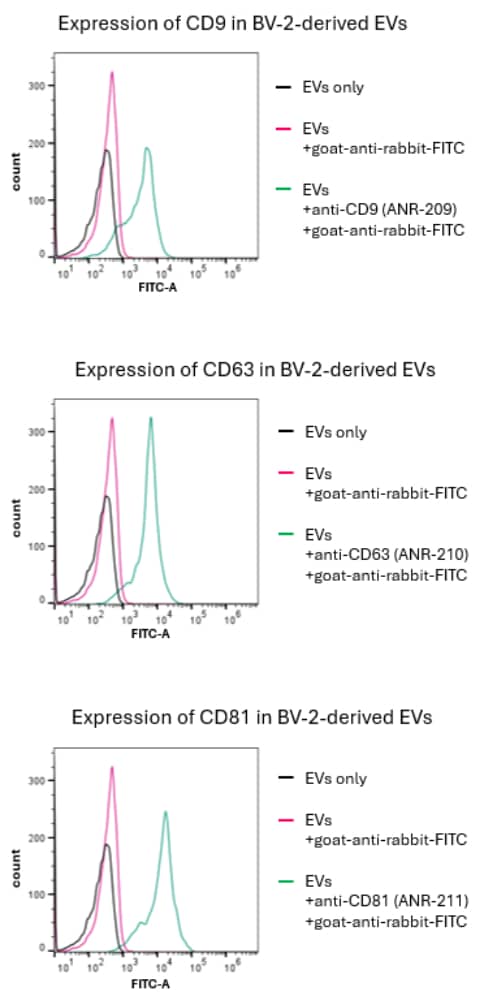
The Clinical Potential of Extracellular Vesicles
The clinical implications of EVs research – as many are now realizing – are vast. The potential of EVs lies in them acting as carriers of therapeutics. EVs bring several advantages as drug carriers: they are naturally equipped with a biocompatible lipid bilayer, can evade immune detection, and are inherently targeted due to their cell-derived surface proteins (20). EVs can also cross the blood-brain barrier, a significant hurdle in treating neurological disorders. Importantly, EVs can be engineered to display specific ligands on their surface to increase their targeting capabilities. This targeted delivery not only increases the therapeutic efficacy but works to also minimize off-target effects and toxicity.
Researchers have already shown that with techniques like electroporation and sonication they can load drugs into EVs by temporarily increasing membrane permeability (21). After being engineered as needed and then administered, EVs deliver their cargo, which includes the active component, through direct fusion with cellular membranes. Alternatively, EVs can interact with target cells via cell-surface receptors, which initiate intracellular signaling.
EVs have shown potential in various therapeutic areas, including oncology, neurology, and regenerative medicine. In cancer therapy, EVs loaded with chemotherapeutic drugs produce efficient delivery to tumor sites and reduced systemic toxicity (22). Additionally, EVs carrying RNA-based therapeutics, such as siRNA, have shown promise in silencing disease-related genes and inducing therapeutic protein expression (23, 24). Similarly, in preclinical animal studies, EVs loaded with anti-inflammatory drugs have shown significant therapeutic outcomes in models of inflammatory diseases (25).
Figure 2. Illustration of EV-mediated cell cross-talk, clearance mechanisms and immune responses. EVs are produced as heterogeneous mixtures of different subpopulations, and they may participate in proximal and distal communication between cells. After entering the systemic circulation, they must avoid elimination organs, such as the liver, lungs and kidneys, as well as immune cells. Their target-tissue efficiency depends on the degree of functionalization and target-cell interaction. Figure and legend are adapted from Herrmann and Wood, Nature Nanotechnology 16, 748-759 (2021) (20).
Challenges to Extracellular Vesicles in the Clinic
Despite their potential, several challenges need to be addressed if we want to fully harness the power and flexibility of EVs as drug delivery platforms. The heterogeneity of EVs population poses a major challenge in their isolation and characterization. Developing standardized methods for EVs production, purification, and quality control is going to be crucial for clinical translation (26).
Another challenge lies in scaling up the production of EVs while adhering to Good Manufacturing Process (GMP)-like conditions. Current isolation methods, such as differential ultracentrifugation and size-exclusion chromatography, are labor-intensive and not easily scalable. Advances in biomanufacturing techniques are needed to produce EVs at a scale suitable for clinical applications (27, 28).
Future research will also need to better understand the biodistribution and pharmacokinetics of EVs in vivo. Comprehensive studies on how EVs distribute within the body, their half-life, and clearance mechanisms will be invaluable when optimizing their design and application (29).
A Powerful Tool from Bench to Bedside
EVs are gathering momentum thanks to their intrinsic properties, relative ease-of-use, and the ability to help us answer question from both an academic and a clinical perspective. As the field continues to grow, the standardization of methods and a focus on personalized medicine will allow more researchers to take advantage of the full potential of EVs, opening up even more areas for discovery. It’s our aim to support you with in-house made and tested reagents you can rely on, as you venture out to take each new opportunity in this exciting and evolving field.
References
1. P. Wolf, The Nature and Significance of Platelet Products in Human Plasma. British Journal of Haematology 13, 269–288 (1967). DOI: https://doi.org/10.1111/j.1365-2141.1967.tb08741.x.
2. N. Crawford, The presence of contractile proteins in platelet microparticles isolated from human and animal platelet-free plasma. Br J Haematol 21, 53–69 (1971). DOI: https://doi.org/10.1111/j.1365-2141.1971.tb03416.x.
3. E. G. Trams, C. J. Lauter, N. Salem, U. Heine, Exfoliation of membrane ecto-enzymes in the form of micro-vesicles. Biochim Biophys Acta 645, 63–70 (1981). DOI: https://doi.org/10.1016/0005-2736(81)90512-5.
4. R. M. Johnstone, M. Adam, J. R. Hammond, L. Orr, C. Turbide, Vesicle formation during reticulocyte maturation. Association of plasma membrane activities with released vesicles (exosomes). J Biol Chem 262, 9412–9420 (1987).
5. K. W. Witwer, C. Théry, Extracellular vesicles or exosomes? On primacy, precision, and popularity influencing a choice of nomenclature. J Extracell Vesicles 8, 1648167 (2019). DOI: https://doi.org/10.1080/20013078.2019.1648167.
6. G. Raposo, H. W. Nijman, W. Stoorvogel, R. Liejendekker, C. V. Harding, C. J. Melief, H. J. Geuze, B lymphocytes secrete antigen-presenting vesicles. J Exp Med 183, 1161–1172 (1996). DOI: https://doi.org/10.1084/jem.183.3.1161.
7. J. Ratajczak, K. Miekus, M. Kucia, J. Zhang, R. Reca, P. Dvorak, M. Z. Ratajczak, Embryonic stem cell-derived microvesicles reprogram hematopoietic progenitors: evidence for horizontal transfer of mRNA and protein delivery. Leukemia 20, 847–856 (2006). DOI: https://doi.org/10.1038/sj.leu.2404132.
8. H. Valadi, K. Ekström, A. Bossios, M. Sjöstrand, J. J. Lee, J. O. Lötvall, Exosome-mediated transfer of mRNAs and microRNAs is a novel mechanism of genetic exchange between cells. Nat Cell Biol 9, 654–659 (2007). DOI: https://doi.org/10.1038/ncb1596.
9. J. Skog, T. Würdinger, S. van Rijn, D. H. Meijer, L. Gainche, M. Sena-Esteves, W. T. Curry, B. S. Carter, A. M. Krichevsky, X. O. Breakefield, Glioblastoma microvesicles transport RNA and proteins that promote tumour growth and provide diagnostic biomarkers. Nat Cell Biol 10, 1470–1476 (2008). DOI: https://doi.org/10.1038/ncb1800.
10. R. A. Dragovic, C. Gardiner, A. S. Brooks, D. S. Tannetta, D. J. P. Ferguson, P. Hole, B. Carr, C. W. G. Redman, A. L. Harris, P. J. Dobson, P. Harrison, I. L. Sargent, Sizing and phenotyping of cellular vesicles using Nanoparticle Tracking Analysis. Nanomedicine 7, 780–788 (2011). DOI: https://doi.org/10.1016/j.nano.2011.04.003.
11. C. Théry, K. W. Witwer, E. Aikawa, et al., Minimal information for studies of extracellular vesicles 2018 (MISEV2018): a position statement of the International Society for Extracellular Vesicles and update of the MISEV2014 guidelines. J Extracell Vesicles 7, 1535750 (2018). DOI: https://doi.org/10.1080/20013078.2018.1535750.
12. C. Théry, L. Zitvogel, S. Amigorena, Exosomes: composition, biogenesis and function. Nat Rev Immunol 2, 569–579 (2002). DOI: https://doi.org/10.1038/nri855.
13. A. Słomka, T. Mocan, B. Wang, I. Nenu, S. K. Urban, M. A. Gonzalez-Carmona, I. G. H. Schmidt-Wolf, V. Lukacs-Kornek, C. P. Strassburg, Z. Spârchez, M. Kornek, EVs as Potential New Therapeutic Tool/Target in Gastrointestinal Cancer and HCC. Cancers 12, 3019 (2020). DOI: https://doi.org/10.3390/cancers12103019.
14. Robbins, P. D., & Morelli, A. E. (2014). Regulation of immune responses by extracellular vesicles. Nature Reviews Immunology, 14(3), 195-208.
15. Peinado, H., Alečković, M., Lavotshkin, S., Matei, I., Costa-Silva, B., Moreno-Bueno, G., … & Lyden, D. (2012). Melanoma exosomes educate bone marrow progenitor cells toward a pro-metastatic phenotype through MET. Nature Medicine, 18(6), 883-891.
16. Raposo, G., & Stoorvogel, W. (2013). Extracellular vesicles: exosomes, microvesicles, and friends. The Journal of Cell Biology, 200(4), 373-383
17. M. Demory Beckler, J. N. Higginbotham, J. L. Franklin, A.-J. Ham, P. J. Halvey, I. E. Imasuen, C. Whitwell, M. Li, D. C. Liebler, R. J. Coffey, Proteomic analysis of exosomes from mutant KRAS colon cancer cells identifies intercellular transfer of mutant KRAS. Mol Cell Proteomics 12, 343–355 (2013). DOI: https://doi.org/10.1074/mcp.M112.022806.
18. B. T. Pan, K. Teng, C. Wu, M. Adam, R. M. Johnstone, Electron microscopic evidence for externalization of the transferrin receptor in vesicular form in sheep reticulocytes. J Cell Biol 101, 942–948 (1985). DOI: https://doi.org/10.1083/jcb.101.3.942.
19. J. Jiang, J. Li, X. Zhou, X. Zhao, B. Huang, Y. Qin, Exosomes Regulate the Epithelial–Mesenchymal Transition in Cancer. Front Oncol 12, 864980 (2022). DOI: https://doi.org/10.3389/fonc.2022.864980.
20. I. K. Herrmann, M. J. A. Wood, Extracellular vesicles as a next-generation drug delivery platform | Nature Nanotechnology. 16, 748-759 (2021). DOI: https://doi.org/10.1038/s41565-021-00931-2
21. X. Luan, K. Sansanaphongpricha, I. Myers, H. Chen, H. Yuan, D. Sun, Engineering exosomes as refined biological nanoplatforms for drug delivery. Acta Pharmacol Sin 38, 754–763 (2017). DOI: https://doi.org/10.1038/aps.2017.12.
22. I. Keklikoglou, C. Cianciaruso, E. Güç, M. L. Squadrito, L. M. Spring, S. Tazzyman, L. Lambein, A. Poissonnier, G. B. Ferraro, C. Baer, A. Cassará, A. Guichard, M. L. Iruela-Arispe, C. E. Lewis, L. M. Coussens, A. Bardia, R. K. Jain, J. W. Pollard, M. De Palma, Chemotherapy elicits pro-metastatic extracellular vesicles in breast cancer models. Nat Cell Biol 21, 190–202 (2019). DOI: https://doi.org/10.1038/s41556-018-0256-3.
23. Y. Rong, Z. Wang, P. Tang, J. Wang, C. Ji, J. Chang, Y. Zhu, W. Ye, J. Bai, W. Liu, G. Yin, L. Yu, X. Zhou, W. Cai, Engineered extracellular vesicles for delivery of siRNA promoting targeted repair of traumatic spinal cord injury. Bioact Mater 23, 328–342 (2022). DOI: https://doi.org/10.1016/j.bioactmat.2022.11.011.
24. J. Sanz-Ros, C. Mas-Bargues, N. Romero-García, J. Huete-Acevedo, M. Dromant, C. Borrás, Extracellular Vesicles as Therapeutic Resources in the Clinical Environment. Int J Mol Sci 24, 2344 (2023). DOI: https://doi.org/10.3390/ijms24032344.
25. K. Lou, H. Luo, X. Jiang, S. Feng, Applications of emerging extracellular vesicles technologies in the treatment of inflammatory diseases. Front. Immunol. 15 (2024). DOI: https://doi.org/10.3389/fimmu.2024.1364401.
26. L. Cabeza, G. Perazzoli, M. Peña, A. Cepero, C. Luque, C. Melguizo, J. Prados, Cancer therapy based on extracellular vesicles as drug delivery vehicles. Journal of Controlled Release 327, 296–315 (2020). DOI: https://doi.org/10.1016/j.jconrel.2020.08.018.
27. E. Rohde, K. Pachler, M. Gimona, Manufacturing and characterization of extracellular vesicles from umbilical cord–derived mesenchymal stromal cells for clinical testing. Cytotherapy 21, 581–592 (2019). DOI: https://doi.org/10.1016/j.jcyt.2018.12.006.
28. M. Zipkin, Exosome redux. Nature Biotechnology 37, 1395–1400 (2019). DOI: https://doi.org/10.1038/s41587-019-0326-5.
29. H. Clemmens, D. W. Lambert, Extracellular vesicles: translational challenges and opportunities. Biochem Soc Trans 46, 1073–1082 (2018). DOI: https://doi.org/10.1042/BST20180112.