Introducing our collection of fluorophore-conjugated extracellular antibodies, designed to streamline and enhance your ability to detect metabolite transporters in live cell samples. With fluorescent-conjugated antibodies that specifically target only the extracellular portion of a protein, you have new opportunities to explore intricate pathways across multiple biological fields.
Fluorophore-Conjugated Extracellular Antibodies Simplify Complexity
Traditional immunofluorescence techniques often rely on indirect methods, which use primary antibodies followed by secondary antibodies conjugated to fluorophores. While effective, this approach introduces complexity, the potential for non-specific binding, and increased variability in results. Direct immunofluorescence, by contrast, uses primary antibodies directly conjugated to fluorophores and offer a simpler, more efficient alternative.
In addition to reducing the number of steps required and minimizing the potential for errors and inconsistencies, directly conjugated extracellular antibodies are ideal for live-cell assays as they allow you to maintain cell viability and offer real-time insights. This method can significantly reduce non-specific binding, so you achieve cleaner, more robust data. Direct conjugation to a fluorophore also boosts your research efficiency by speeding up experimental procedures, saving time, and reducing costs associated with additional reagents and steps needed in indirect methods. These benefits really stand out in applications like live cell flow cytometry where both complexity and scope for error are high.
At Alomone Labs, we’ve developed hundreds of fluorophore-conjugated extracellular antibodies that can be conjugated to a range of fluorophores, including ATTO Fluor (488 to 647 nm), fluorescein isothiocyanate (FITC), phycoerythrin (PE), and allophycocyanin (APC). This variety means you can customize your multiplex assays to meet your specific research needs and objectives. You can also request a custom conjugation if you need something that isn’t listed on the catalog.
A notable application of our antibodies is an ability to distinguish and track simultanously distinct metabolite transporters in live cells using different fluorophores. For instance:
- Anti-GLUT1 (extracellular)-FITC Antibody (#AGT-041-F), conjugated to FITC
- Anti-MCT1 (SLC16A1) (extracellular)-APC Antibody (#AMT-011-APC), linked to APC.
- Anti-Human ASCT2/SLC1A5 (extracellular)-PE Antibody (#ANT-083-PE), conjugated to PE.
Each of these three transporters play a crucial role in the metabolic programs of T cells. We’ll take a closer look at the antibodies and their targets in the context of T cell function within the tumor microenvironment (TME) to illustrate the utility of our technology. Keep in mind they represent just a fraction of the broader applications possible with our full antibody collection.
Benefits to T cell Metabolism Research
Being able to visualize multiple transporters simultaneously is incredibly valuable when studying the metabolic behavior of T cells. T cells rely on efficient metabolic programming to function effectively, especially in the metabolically hostile TME, and growing research suggest that nutrients are essential for T cell proper activation (1). The TME, characterized by nutrient scarcity, high levels of waste products, and hypoxia, can impose significant constraints on T cell activity. With this in mind, let’s delve a little deeper into our transporters of interest.
Glucose Uptake and GLUT1: Glucose transporter 1 (GLUT1) managing basal-level glucose uptake into many tissues. GLUT1, which contains 12 membrane-spanning domains with both amino and carboxyl termini oriented intracellularly, is widely expressed, being most abundant in fibroblasts, erythrocytes, and endothelial cells. It is essential for T cell activation and proliferation. In the TME, cancer cells consume vast amounts of glucose, limiting its availability for T cells. This glucose depletion can impair T cell function, reducing their proliferation and cytokine production (2). Human memory T cells expressing higher levels of GLUT1 show stronger effector memory T cell phenotypes (3). The ability to use an immunoassay to follow GLUT1 dynamics in live cells, means researchers can better understand how glucose uptake influences T cell behavior and identify potential interventions to enhance T cell function.
Lactate Export and MCT1: Monocarboxylate transporter 1 (MCT1) facilitates the transport of key metabolic intermediates such as pyruvate, lactate, and ketone bodies across the plasma membrane. Comprised of 12 transmembrane helices with intracellular C- and N-termini, MCT1 functions predominantly to help the proton-linked transport of L-lactate. This can result in either influx or efflux of lactic acid, depending on the prevailing intracellular and extracellular substrate concentrations and the pH gradient across the plasma membrane. Elevated lactate levels in the TME can inhibit T cell function and promote regulatory T cell survival, which suppresses immune responses (4, 5). Studying MCT1 in live cells helps us elucidate the mechanisms by which T cells manage metabolic waste and maintain their effector functions in acidic environments.
Glutamine Uptake and ASCT2: Alanine/serine/cysteine transporter 2 (ASCT2) functions as a high-affinity glutamine transporter. ASCT2 is vital for glutamine uptake to support the tricarboxylic acid cycle and biosynthetic processes necessary for T cell activation. It also serves as a Na+-dependent transporter for alanine, serine, and cysteine. Studies have shown that inadequate glutamine availability in the TME can impair T cell responses and promote tumor progression (6). By tracking ASCT2 activity in live cells, researchers can gain insights into how glutamine metabolism affects T cell function and explore how nutrient availability impacts T cells in the TME.
In our labs, we have developed an immunometabolic T cell panel for flow cytometry using our three focus antibodies (Figure 1) to help researchers begin to address some of these topics.
Alomone Labs’ Immunometabolic T cell Panel For Live Flow Cytometry
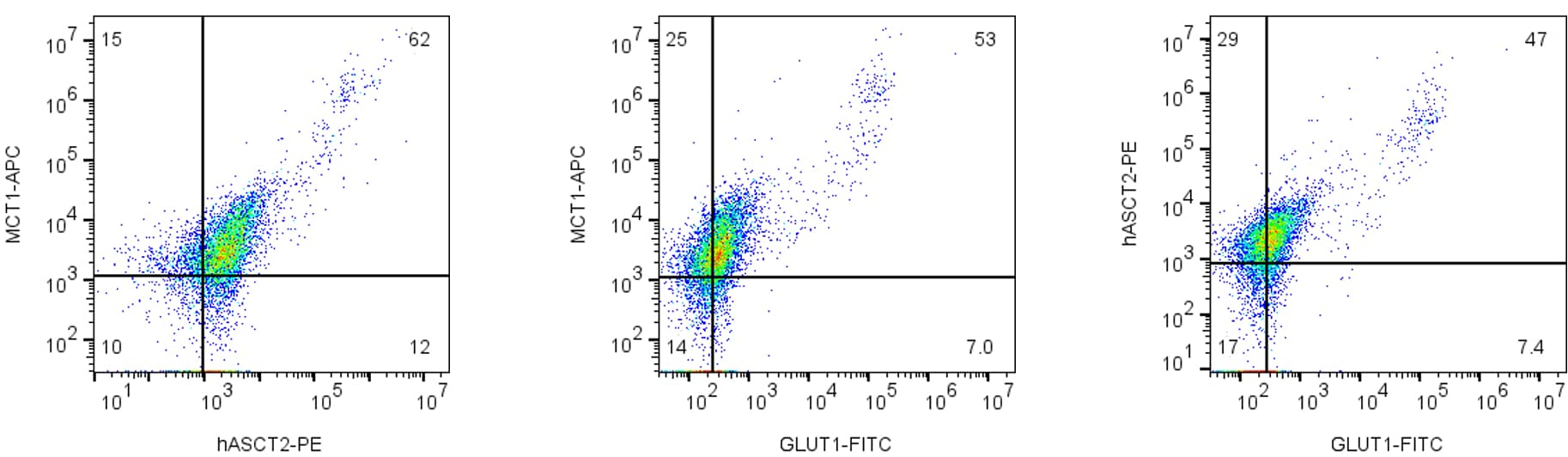
Figure 1. Multicolor flow cytometry analysis for ASCT2, MCT1, and GLUT1 markers in live intact human Jurkat T cells. Jurkat T cells were labeled with different combinations of Anti-Human ASCT2/SLC1A5 (extracellular)-PE Antibody (#ANT-083-PE), Anti-MCT1 (SLC16A1) (extracellular)-APC Antibody (#AMT-011-APC), and Anti-GLUT1 (extracellular)-FITC Antibody (#AGT-041-F).
Enhancing Immunotherapy Research
Understanding the metabolic constraints imposed by the TME on T cells is critical for advancing cancer immunotherapy, and our fluorescent-conjugated extracellular antibodies can be used to examine metabolic pathways integral for T cell function. This knowledge can inform the development approaches to enhance T cell activity and improve the efficacy of immunotherapies.
For instance, targeting GLUT1 to boost glucose uptake or mitigating lactate accumulation through MCT1 could help T cells maintain their effector functions even in the nutrient-depleted and acidic conditions of the TME (4, 7). Promoting GLUT1 expression can enhance T cell proliferation and cytokine production, thereby improving their anti-tumor efficacy (8). Additionally, ensuring adequate glutamine supply through ASCT2 could enhance T cell proliferation and anti-tumor activity (6).
Studies have shown that metabolic interventions can significantly impact T cell function within the TME. High lactate levels in the TME rewire pyruvate metabolism in CD8+ T cells, leading to reduced succinate secretion and impaired cytotoxic function (9). Lactate uptake by CD8+ T cells causes intracellular acidification and impairs signaling of nuclear factor of activated T cells. Reducing lactate abundance or uptake may potentiate CD8+ T cells while suppressing regulatory T cells function in glycolytic tumors (10).
Research has now even extended into how dietary interventions can modulate the expression and function of nutrient transporters critical for T cell metabolism. For instance, intermittent fasting has been found to protect against CNS autoimmunity by altering the gut microbiota, which in turn affects systemic immunity, including T cell function (11). Additionally, microbiota-derived short-chain fatty acids can enhance the memory potential of antigen-activated CD8+ T cells, linking diet to improved T cell responses (12).
Broader Applications
The versatility of these conjugated extracellular antibodies extends beyond T cell metabolism and will have positive effects on multiple fields of cellular and molecular biology. Because these antibodies enable real-time, multicolor detection of different transporters and receptors on live cells, they provide invaluable data on cellular dynamics and interactions. For example, researchers studying neuronal activity, stem cell differentiation, or immune responses can benefit from this technology by gaining a clearer understanding of how cells regulate nutrient transport and signaling pathways in real-time.
In neuroscience, the ability to visualize multiple transporters simultaneously can shed light on the metabolic requirements of neurons and glial cells, advancing our understanding of neurodegenerative diseases and brain metabolism. Stem cell researchers can use these antibodies to monitor the metabolic changes that occur during differentiation and proliferation to help us look into how metabolic pathways influence stem cell fate decisions. In oncology, cancer researchers can investigate how different cancer cell types alter their metabolism to survive and proliferate in various microenvironments. This information could lead to the development of novel metabolic therapies targeting cancer cells while sparing normal cells.
A Bright Future for Research
This collection of conjugated extracellular antibodies is a valuable set of tools for anyone studying cellular metabolism. By enabling the precise and simultaneous detection of multiple metabolite transporters in live cells, researchers using tools like these are contributing to our growing knowledge as well as the potential for more effective therapies and interventions. With robust and specific antibodies conjugated directly to a range of fluorophore options, the future of research is truly bright.
References
1. J. L. Raynor, H. Chi, Nutrients: Signal 4 in T cell immunity. Journal of Experimental Medicine 221, e20221839 (2024). DOI: https://doi.org/10.1084/jem.20221839.
2. C. M. Cham, G. Driessens, J. P. O’Keefe, T. F. Gajewski, Glucose deprivation inhibits multiple key gene expression events and effector functions in CD8+ T cells. Eur J Immunol 38, 2438–2450 (2008). DOI: https://doi.org/10.1002/eji.200838289.
3. G. Cretenet, I. Clerc, M. Matias, S. Loisel, M. Craveiro, L. Oburoglu, S. Kinet, C. Mongellaz, V. Dardalhon, N. Taylor, Cell surface Glut1 levels distinguish human CD4 and CD8 T lymphocyte subsets with distinct effector functions. Sci Rep 6, 24129 (2016). DOI: https://doi.org/10.1038/srep24129.
4. K. Fischer, P. Hoffmann, S. Voelkl, N. Meidenbauer, J. Ammer, M. Edinger, E. Gottfried, S. Schwarz, G. Rothe, S. Hoves, K. Renner, B. Timischl, A. Mackensen, L. Kunz-Schughart, R. Andreesen, S. W. Krause, M. Kreutz, Inhibitory effect of tumor cell-derived lactic acid on human T cells. Blood 109, 3812–3819 (2007). DOI: https://doi.org/10.1182/blood-2006-07-035972.
5. A. Sugiura, J. C. Rathmell, Metabolic Barriers to T Cell Function in Tumors. The Journal of Immunology 200, 400–407 (2018). DOI: https://doi.org/10.4049/jimmunol.1701041.
6. R. Wang, C. P. Dillon, L. Z. Shi, S. Milasta, R. Carter, D. Finkelstein, L. L. McCormick, P. Fitzgerald, H. Chi, J. Munger, D. R. Green, The transcription factor Myc controls metabolic reprogramming upon T lymphocyte activation. Immunity 35, 871–882 (2011). DOI: https://doi.org/10.1016/j.immuni.2011.09.021.
7. C. M. Murray, R. Hutchinson, J. R. Bantick, G. P. Belfield, A. D. Benjamin, D. Brazma, R. V. Bundick, I. D. Cook, R. I. Craggs, S. Edwards, L. R. Evans, R. Harrison, E. Holness, A. P. Jackson, C. G. Jackson, L. P. Kingston, M. W. D. Perry, A. R. J. Ross, P. A. Rugman, S. S. Sidhu, M. Sullivan, D. A. Taylor-Fishwick, P. C. Walker, Y. M. Whitehead, D. J. Wilkinson, A. Wright, D. K. Donald, Monocarboxylate transporter MCT1 is a target for immunosuppression. Nat Chem Biol 1, 371–376 (2005). DOI: https://doi.org/10.1038/nchembio744.
8. S. R. Jacobs, C. E. Herman, N. J. Maciver, J. A. Wofford, H. L. Wieman, J. J. Hammen, J. C. Rathmell, Glucose uptake is limiting in T cell activation and requires CD28-mediated Akt-dependent and independent pathways. J Immunol 180, 4476–4486 (2008). DOI: https://doi.org/10.4049/jimmunol.180.7.4476.
9. I. Elia, J. H. Rowe, S. Johnson, S. Joshi, G. Notarangelo, K. Kurmi, S. Weiss, G. J. Freeman, A. H. Sharpe, M. C. Haigis, Tumor cells dictate anti-tumor immune responses by altering pyruvate utilization and succinate signaling in CD8+ T cells. Cell Metabolism 34, 1137-1150.e6 (2022). DOI: https://doi.org/10.1016/j.cmet.2022.06.008.
10. A. Brand, K. Singer, G. E. Koehl, M. Kolitzus, G. Schoenhammer, A. Thiel, C. Matos, C. Bruss, S. Klobuch, K. Peter, M. Kastenberger, C. Bogdan, U. Schleicher, A. Mackensen, E. Ullrich, S. Fichtner-Feigl, R. Kesselring, M. Mack, U. Ritter, M. Schmid, C. Blank, K. Dettmer, P. J. Oefner, P. Hoffmann, S. Walenta, E. K. Geissler, J. Pouyssegur, A. Villunger, A. Steven, B. Seliger, S. Schreml, S. Haferkamp, E. Kohl, S. Karrer, M. Berneburg, W. Herr, W. Mueller-Klieser, K. Renner, M. Kreutz, LDHA-Associated Lactic Acid Production Blunts Tumor Immunosurveillance by T and NK Cells. Cell Metab 24, 657–671 (2016). DOI: https://doi.org/10.1016/j.cmet.2016.08.011.
11. F. Cignarella, C. Cantoni, L. Ghezzi, A. Salter, Y. Dorsett, L. Chen, D. Phillips, G. M. Weinstock, L. Fontana, A. H. Cross, Y. Zhou, L. Piccio, Intermittent Fasting Confers Protection in CNS Autoimmunity by Altering the Gut Microbiota. Cell Metab 27, 1222-1235.e6 (2018). DOI: https://doi.org/10.1016/j.cmet.2018.05.006.
12. A. Bachem, C. Makhlouf, K. J. Binger, D. P. de Souza, D. Tull, K. Hochheiser, P. G. Whitney, D. Fernandez-Ruiz, S. Dähling, W. Kastenmüller, J. Jönsson, E. Gressier, A. M. Lew, C. Perdomo, A. Kupz, W. Figgett, F. Mackay, M. Oleshansky, B. E. Russ, I. A. Parish, A. Kallies, M. J. McConville, S. J. Turner, T. Gebhardt, S. Bedoui, Microbiota-Derived Short-Chain Fatty Acids Promote the Memory Potential of Antigen-Activated CD8+ T Cells. Immunity 51, 285-297.e5 (2019). DOI: https://doi.org/10.1016/j.immuni.2019.06.002.
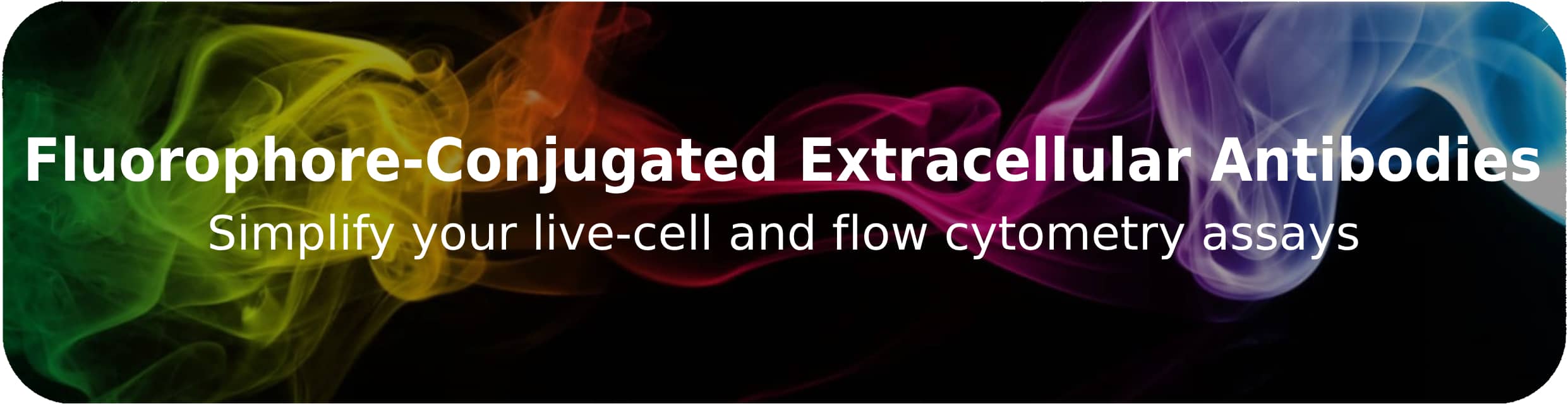