When you need to look at ion channels and an antibody just won’t do, why not try a fluorescent probe attached to some scorpion venom?
You already know what ion channels do and how important they are in both regular physiology and medicine, so we’re not going to bore you with all the textbook definitions. What is interesting, though, is the possibility of using natural toxins to visualize and probe the function of ion channels.
Understanding how things like voltage-gated sodium (Nav) and potassium (Kv) channels function is obviously important for a huge amount of research. Alongside understanding function is being able to describe the distribution of these channels within tissues. You’d probably use an immunoassay, with an antibody to bind the channel you’re looking at, which you’d then detect with a probe conjugated to that primary antibody or a secondary antibody with a microscope. Pretty standard stuff.
The unique greatness of toxins
But antibodies are fairly large proteins and the simple act of antibody binding can affect ion channel function 1,2. Also, you usually need to fix your sample when using an antibody. So, what else is there?
Well, instead of relying on the good old antibody, nature has provided us with a suite of natural toxins that make some of the best neurobiology research tools. Some of the features you can expect from toxins in your ion channel research include
- Exquisite selectivity
- High potency and specificity
- Nanomolar or picomolar affinity
- You can use them in living tissues, so no need to fix
- The ability to distinguish between even similar varieties of ion channels
- The ability to modulate channel function in multiple ways
Toxin peptides already get a lot of use in electrophysiology because of these fantastic properties. But while patch clamping is a gold standard when it comes to monitoring channel activity, it doesn’t offer anything for looking at channel distribution.
Toxin + probe
But here you have toxins: a highly diverse group of peptides that can target specific types of ion channels with incredible accuracy. And they’re ready to bind your research target. So how do you keep track of a toxin and use it to understand channel distribution?
You conjugate it to a probe.
With the toxin already doing the challenging work of targeting the channel, all you now need is a probe, like an ATTO-Fluor or FITC, for example, to attach to the toxin. Toxins can also be conjugated to biotin. The result is a small, specific peptide that binds your ion channel without the need to fix samples or the various issues that go along with using a large protein like an antibody.
These are remarkable tools for doing even more detailed research into ion channel function and distribution but sadly labeled toxins don’t receive the attention they deserve.
Thankfully, some people are putting toxins to clever use. You can see some wonderful examples of labeled toxins from scientists who made a chimeric construct of a fluorescent protein fused to a Kv channel scorpion toxin3. Similarly, others have used Cy5 conjugated to saxitoxin, the paralytic shellfish poison4, or various Alexa dyes conjugated to the scorpion toxins, tityustoxin (TsTX), and toxin-gamma (TiTX-g)5, to research Nav channels.
If you want to read a little more about methods to conjugate probes to toxins, you should go and have a look at our article on that very subject.
The great thing is that toxins continue to receive attention into how they interact with ion channels6, meaning we’ll continue to come up with new and exciting ways to use labeled varieties of these toxins in ion channel research.
Making visuals with venom toxin
As you can see below, with a toxin labeled with a fluorescent probe you can create beautiful and revealing microscopy images. Using a labeled toxin like this also significantly expands your multiplex options, opening up the possibility to use these alongside antibodies for incredibly detailed datasets.
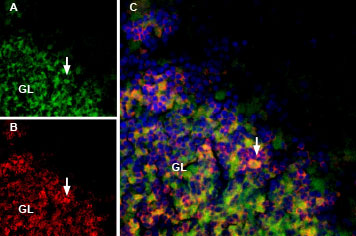
Rat brain sections incubated with MmTx1 Toxin-ATTO Fluor-488 (#STM-550-AG) at a concentration of 25 nM. The sections were then incubated with Anti-GABA(A) α1 Receptor (extracellular) Antibody (#AGA-001), followed by donkey anti-rabbit labeled with Cy3 (red). A: MmTx1 Toxin-ATTO Fluor-488 binding (green) appears in patches within the granule layer (GL), (arrows). B: GABA(A) α1 receptor immunostaining appears in profiles of GL neurons (arrow). C. Merge of the two images demonstrates variable colocalization. DAPI is used as the counterstain.
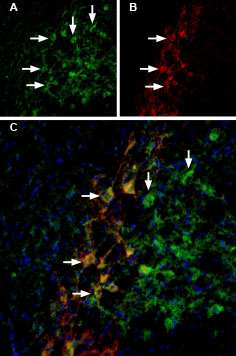
Rat brain sections were first incubated with 33 nM Tertiapin-Q-ATTO Fluor-488 (#STT-170-AG), (green). The same sections were incubated with Guinea pig Anti-GIRK2 (Kir3.2) Antibody (#AGP-013). A: Tertiapin-Q-ATTO Fluor-488 binding appears in profiles of substantia nigra pars compacta (SNC) neurons (arrows). B: GIRK2 staining (red) is detected in profiles of SNC neurons (arrows). C: Merge of the two images demonstrates co-staining of GIRK2 channels by Tertiapin-Q-ATTO Fluor-488 and by Guinea pig Anti-GIRK2 (Kir3.2) Antibody. DAPI staining (blue) is used to stain nuclei.
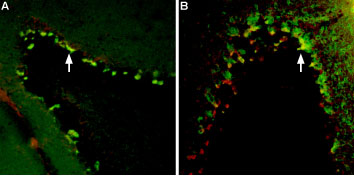
A: Multiplex Staining of free-floating mouse brain sections using Tityustoxin-Kα-ATTO Fluor-594 (#STT-360-AR) (red). Sections were then stained using Anti-KV1.2 (KCNA2) Antibody (#APC-010) (1:200) followed by goat anti-rabbit-AlexaFluor-488 (green). Tityustoxin-Kα-ATTO Fluor-594 and KV1.2 are both detected in the pinceau structures (yellow staining, arrow) of the cerebellum. B: Same brain sections as in A were labeled with Tityustoxin-Kα-ATTO Fluor-594 (red) followed by anti-Parvalbumin. Tityustoxin-Kα-ATTO Fluor-594 (red) labeled the pinceau structures (arrow) of the cerebellum. Parvalbumin stained not only the pinceau but also the soma of Purkinje cells. Co-localization between the two is depicted in yellow.
Sound interesting?
We’re not saying you should ditch your antibodies in favor of labeled toxins, right now. But, if you’re looking at ion channels, you should probably come and give labeled toxins a try if you want to use something that’s both super potent and reliable. Put a little more sting into your science.
References
- Schofield, G. G., Puhl, H. L. & Ikeda, S. R. Properties of wild-type and fluorescent protein-tagged mouse tetrodotoxin-resistant sodium channel (NaV1.8) heterologously expressed in rat sympathetic neurons. J. Neurophysiol. 99, (2008).
- Maue, R. A. Understanding ion channel biology using epitope tags: Progress, pitfalls, and promise. Journal of Cellular Physiology vol. 213 (2007).
- Kuzmenkov, A. I. et al. Fluorescent protein-scorpion toxin chimera is a convenient molecular tool for studies of potassium channels. Sci. Rep. 6, 1–10 (2016).
- Ondrus, A. E. et al. Fluorescent saxitoxins for live cell imaging of single voltage-gated sodium ion channels beyond the optical diffraction limit. Chem. Biol. 19, 902–912 (2012).
- Massensini, A. R. et al. Tracking sodium channels in live cells: Confocal imaging using fluorescently labeled toxins. J. Neurosci. Methods 116, 189–196 (2002).
- Jiang, D. et al. Structural basis for voltage-sensor trapping of the cardiac sodium channel by a deathstalker scorpion toxin. Nat. Commun. 12, (2021).