Overview
- Peptide (C)NGVPESTSTDTPPDIDLHN, corresponding to amino acid residues 392-410 of human Kir2.1 (Accession P48049). Intracellular, C-terminal part.
- Western blot analysis of rat heart (lanes 1 and 3) and rat brain (lanes 2 and 4) membranes:1,2. Anti-Kir2.1/KCNJ2 Antibody (#APC-026), (1:200).
3,4. Anti-Kir2.1/KCNJ2 Antibody, preincubated with Kir2.1/KCNJ2 Blocking Peptide (#BLP-PC026).
- Transfected HEK 293 cell lysate (Preisig Muller, R. et al. (2002) Proc. Natl. Acad. Sci. U.S.A. 99, 7774.).
- Rat brain sections.
- Mouse ventricular myocytes (1:200) (Clark, R.B. et al. (2001) J. Physiol. 537, 979.).
- Human bladder urothelial cells (BUC) (Sun, Y. et al. (2007) Am. J. Physiol. 292, C106.).
- Kubo, Y. et al. (1993) Nature 362, 127.
- Nichols, C.G. et al. (1996) Circ. Res. 78, 1.
- Plaster, N.M. et al. (2001) Cell 105, 511.
Kir2.1 is a member of the family of inward rectifying K+ channels. The family includes 15 members that are structurally and functionally different from the voltage-dependent K+ channels.1
The family’s topology consists of two transmembrane domains that flank a single and highly conserved pore region with intracellular N- and C-termini. As is the case for the voltage-dependent K+ channels the functional unit for the Kir channels is composed of four subunits that can assemble as either homo- or heterotetramers.
Kir channels are characterized by a K+ efflux that is limited by depolarizing membrane potentials thus making them essential for controlling resting membrane potential and K+ homeostasis.
Kir2.1 is a member of the Kir2.x subfamily that includes four members (Kir2.1- Kir2.4) that are characterized by strong inward rectification and high constitutive activity.
Kir2.1 is expressed in a variety of tissues including the heart, brain, vascular smooth muscle cells and skeletal muscles.
In the heart, Kir2.1 is a molecular component of the IK1 current that is responsible for setting the resting membrane potential, preventing membrane hyperpolarization due to Na+ pump activity, influencing propagation velocity, altering the electrical space constant, and promoting late phase repolarization.2 In fact, mutations in Kir2.1 channels have been linked to a form of long QT syndrome (LQT7) known as Andersen's syndrome that is characterized by cardiac arrhythmias, periodic paralysis, and dysmorphic features.3
Application key:
Species reactivity key:
Alomone Labs is pleased to offer a highly specific antibody directed against an epitope of human Kir2.1 channel. Anti-Kir2.1/KCNJ2 Antibody (#APC-026) can be used in western blot, immunoprecipitation, immunocytochemistry, immunohistochemistry, and flow cytometry applications. It has been designed to recognize Kir2.1 from human, rat, and mouse samples.
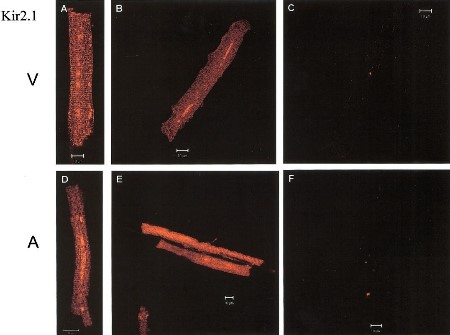
Expression of Kir2.1 in canine myocytes.
Immunocytochemical staining of canine myocytes using Anti-Kir2.1/KCNJ2 Antibody (#APC-026). Kir2.1 staining (green) is detected in both ventricle (V) and atria (A). Kir2.1 staining is eradicated when antibody is incubated with the peptide antigen (C and F).Adapted from Melnyk, P. et al. (2002) Am. J. Physiol. 283, 1123. with permission of The American Physiological Society.
Applications
Citations
- Expression of Kir2.1 in human U-251 MG cells.A. Immunocytochemical staining of human astrocytoma U-251 MG cells expressing WT or K346T channels using Anti-Kir2.1/KCNJ2 Antibody (#APC-026) (red). WT channels are localized in perinuclear vesicles (short arrows in upper panel) and occasionally at plasma membranes (long arrows in upper panel). Mutated channels are mainly expressed at plasma membranes (long arrows in lower panel). B. WB analysis of membrane (MEM) and cytosolic (CYT) proteins derived from WT or K346T Kir2.1-expressing cells using Anti-Kir2.1/KCNJ2 Antibody. D. Densitometric analysis of protein bands from four independent experiments (mean ± SEM, ∗P < 0.05).
Adapted from Ambrosini, E. et al. (2014) with permission of Oxford University Press. - Expression of Kir2.1 and Kir2.3 channels in Lamina I pacemaker cells.Immunohistochemical staining of rat spinal cord sections using Lamina I pacemaker using Anti-Kir2.1/KCNJ2 Antibody (#APC-026) and Anti-Kir2.3 (KCNJ4) Antibody (#APC-032). Confocal images of representative biocytin-filled pacemaker neurons (red) processed for Kir (green). Merged images demonstrate that immunoreactive puncta for Kir2.1 and Kir2.3 (yellow; inset) are localized to identified pacemaker neurons within lamina I of the neonatal spinal cord (right). Scale bars: 10 μm; inset, 2 μm.
Adapted from Li, J. et al. (2013) with permission of The Society for Neuroscience.
- Human THP-1 cells.
Kim, K.S. et al. (2015) J. Immunol. 195, 3345. - Mouse activated macrophages (BMDM cells).
Moreno, C. et al. (2013) J. Immunol. 191, 6136.
- Transfected HEK 293 cell lysate.
Preisig Muller, R. et al. (2002) Proc. Natl. Acad. Sci. U.S.A. 99, 7774.
- Rat retina sections (1:100).
Li, Q. et al. (2017) Brain Struct. Funct. 222, 813. - Rat dorsal root ganglia and spinal cord sections (1:200).
Murata, Y. et al. (2016) Neurosci. Lett. 617, 59. - Rat spinal cord sections (1:200).
Li, J. et al. (2013) J. Neurosci. 33, 3352. - Rat heart sections (1:20).
Atkinson, A.J. et al. (2013) J. Am. Heart Assoc. 2, e000246.
- HEK 293 transfected cells (1:200).
Murata, Y. et al. (2016) Neurosci. Lett. 617, 59. - Human THP-1 cells (1:200).
Kim, K.S. et al. (2015) J. Immunol. 195, 3345. - Human astrocytoma U-251 MG cells.
Ambrosini, E. et al. (2014) Hum. Mol. Genet. 23, 4875. - Mouse microglia (1:100).
Muessel, M.J. et al. (2013) Glia 61, 1620. - Mouse activated macrophages (BMDM cells).
Moreno, C. et al. (2013) J. Immunol. 191, 6136. - Mouse cardiomyocytes (1:200).
Fujiwara, M. et al. (2011) PLoS One 6, e16734. - Canine myocytes.
Melnyk, P. et al. (2002) Am. J. Physiol. 283, 1123. - Mouse ventricular myocytes (1:200).
Clark, R.B. et al. (2001) J. Physiol. 537.3, 979.
- Human bladder urothelial cells (BUC).
Sun, Y. et al. (2007) Am. J. Physiol. 292, C106.
- Askar, S.F. et al. (2013) Cardiovasc. Res. 97, 171.
- Bonilla, I.M. et al. (2012) J. Appl. Physiol. 113, 1772.
- Hong, M. et al. (2012) J. Biol. Chem. 287, 41258.
- Tajada, S. et al. (2012) J. Physiol. 590, 6075.
- Eberhardt, C. et al. (2011) Glia 59, 697.
- Yamazaki, D. et al. (2011) Am. J. Physiol. 300, C75.
- Pivonkova, H. et al. (2010) Neurochem. Int. 57, 783.
- Bocksteins, E. et al. (2009) Am. J. Physiol. 296, C1271.
- Howorth, P.W. et al. (2009) J. Neurosci. 29, 12855.
- Shan, H. et al. (2009) Br. J. Pharmacol. 158, 1227.
- Hinard, V. et al. (2008) Development 135, 859.
- Narazaki, G. et al. (2008) Circulation 118, 498.
- Vit, J.P. et al. (2008) J. Neurosci. 28, 4861.
- Yanagi, K. et al. (2007) Stem Cells 25, 2712.
- Gavillet, B. et al. (2006) Circ Res. 99, 407.
- Grishin, A. et al. (2006) J. Biol. Chem. 281, 30104.
- Gaborit, N. et al. (2005) Circulation 112, 471.
- Melnyk, P. et al. (2005) Cardiovasc. Res. 65, 104.
- Dhamoon, A.S. et al. (2004) Circ. Res. 94, 1332.
- Malinowska, D.H. et al. (2004) Am. J. Physiol. 286, C495.
- Yan, L. et al. (2004) Diabetes 53, 597.
- Karkanis, T. et al. (2003) Am. J. Physiol. 284, H2325.
- Vicente, R. et al. (2003) J. Biol. Chem. 278, 46307.
- Ennis, I.L. et al. (2002) J. Clin. Invest. 109, 393.
- Giovannardi, S. et al. (2002) J. Biol. Chem. 277, 12158.
- Trepanier-Boulay, V. et al. (2001) Circ Res 89, 437.
- Keren-Raifman, T. et al. (2000) Biochem. Biophys. Res. Commun. 274, 852.