Tissue clearing transforms opaque samples into transparent ones, so you can use antibodies to look inside tissues without having to slice them up. Don’t get us wrong: we’re not against slicing samples up, it’s just that they only tell a partial story. Preserving tissue architecture with tissue clearing, on the other hand, allows capturing the spatial relationships between structures, cells and proteins. Thankfully, there have been a lot of very clever folk over the years who have figured out how to make tissues see-through and accessible to our microscopes.
That means there are a lot of clearing options to choose from. This overview’s here to help. We’ll break down some of the main tissue clearing techniques, highlight where each one excels – and where each one has room for improvement – so you can understand why you might choose one over another. We’ll also pull out some beautiful data so you can see these techniques in action.
Solvent-Based Clearing Techniques
Solvent-based techniques are your go-to rapid clearing options that work by removing lipids with organic solvents. These techniques are great for large samples and whole-organ imaging but can leave you with significant tissue shrinkage and fluorescence quenching.
BABB
Benzyl Alcohol and Benzyl Benzoate (BABB) technique is a brute-force method developed in the late 80s that works by dissolving lipids with organic solvents to remove lipids and other components that scatter light to make them transparent (1). At the end of the process your tissue is clear and retains its structural integrity, which is why BABB is often used for large, lipid-rich samples like organs.
- Mechanism: BABB uses a combination of benzyl alcohol and benzyl benzoate to remove lipids. This process reduces the refractive index (RI) mismatch between the tissue and its surroundings and makes the tissue transparent.
- Application: Best suited for samples where lipid removal isn’t critical to the integrity of the structures of interest, such as in connective tissues or certain organs.
- Pros: Speedy clearing process, so it’s ideal for when you have to quickly prepare large samples for imaging.
- Cons: BABB leads to tissue shrinkage and may damage delicate structures simply due to the how harsh the solvents can be (2, 3).
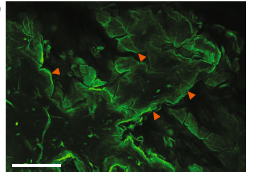
Figure 1. Mouse Dermis Cleared with BABB. 40X confocal imaging of cleared (BABB), uninjured L2G mouse skin whole-mounted with the hypodermis facing upwards (closest to the objective), shows that epidermis (farthest from the objective) can be clearly visualized through the full thickness of the tissue (red arrowheads highlight edges of keratinocyte “sheets” characteristic of epidermal biology. Scale bar = 200 μm. From Foster et al. (2019) (4).
3DISCO and iDISCO
3D Imaging of Solvent-Cleared Organs (3DISCO) and Immunolabeling-enabled DISCO (iDISCO) techniques take this a step further by letting you detect specific proteins with most standard immunoassays (5, 6).
- 3DISCO: Uses a series of dehydration steps with tetrahydrofuran (THF) followed by dibenzyl ether (DBE). At the end, you’re left with transparent tissues that are suitable for whole-organ imaging.
- iDISCO: Involves pre-treating the tissue with methanol and hydrogen peroxide to improve antibody penetration and reduce background fluorescence.
- Pros: Both techniques are quick and great for large tissue volumes. iDISCO’s immunolabeling options makes it especially valuable for looking at protein localization.
- Cons: There’s no way around the fact that harsh solvents can quench fluorescence over time and so limit your options for long-term studies. Tissue shrinkage and potential deformation are also typical concerns (4, 7).
uDISCO
The DISCO techniques work well, but of course have some shortcomings. So, in 2016 the Ultimate 3D Imaging of Solvent-Cleared Organs (uDISCO) came along with the goal to reduce some of the typical drawbacks like tissue shrinkage and fluorescence quenching (8). And it worked. uDISCO is very effective for whole-organ clearing with minimal loss of fluorescence over time.
- Mechanism: Uses a dehydration process followed by a clearing step with a carefully optimized cocktail of organic solvents. This approach significantly minimizes tissue shrinkage, with reported reductions of only about 20–30%, which is far less than earlier solvent-based methods.
- Application: uDISCO is ideal for long-term imaging of large tissues, such as the entire nervous system, where maintaining fluorescence and structural integrity over extended periods is crucial – uDISCO can preserves fluorescent proteins over months.
- Pros: uDISCO gives you excellent fluorescent signal preservation and minimizes tissue shrinkage compared with other solvent-based methods.
- Cons: While uDISCO reduces some of the common issues with solvent-based clearing, it still relies on harsh solvents, which can be challenging to handle and may require specialized equipment and safety precautions (2, 7).
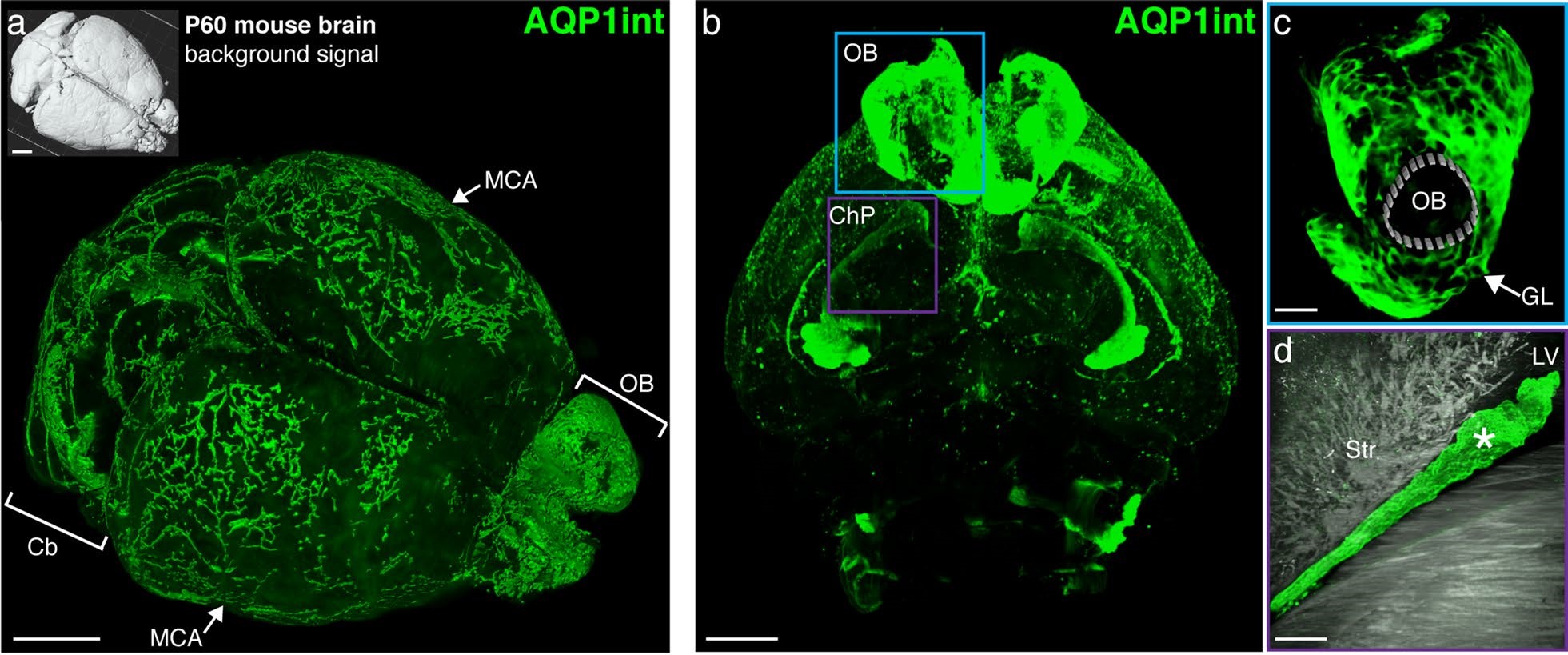
Figure 2. Aquaporin 1 expression in intact mouse brain cleared with uDISCO. a: Mouse brain (P60) cleared by uDISCO and immunolabeled for AQP1 (AQP1int, green, using Alomone Anti-Aquaporin 1 Antibody (#AQP-001)) reveals the vasculature network in the leptomeninges, including the middle cerebral arteries (MCA, arrows). AQP1+ cells also line the subarachnoid cisterns and the olfactory bulb. b: Optical section reveals AQP1+ choroidal epithelial cells and olfactory ensheathing glia cells. From Li et al. (2020) (9).
Aqueous-Based Clearing Techniques
Aqueous-based methods use water-soluble reagents to do the tissue clearing and also manage to preserve structural integrity and endogenous fluorescence. They were developed to be safer, simpler, and more fluorescence-friendly alternatives to solvent-based methods. Aqueous-based clearing methods are perfect for when you need detailed imaging of delicate tissues like the brain – but be prepared to wait as generally need relatively long clearing time. You can divide these into two broad categories: simple immersion and delipidation/hydration methods.
Simple Immersion Methods
Simple immersion methods involve soaking tissues in high RI aqueous solutions to gradually make your samples transparent without extensive tissue deformation. Common reagents used in this include fructose, 2,20-thiodiethanol (TDE), and formamide. Some of the techniques here include (10):
- SeeDB and FRUIT: SeeDB, a fructose-based method, is good for clearing mouse brain samples with minimal tissue deformation, though it’s not practical for whole-organ clearing. To address this, the SeeDB-derived FRUIT method, which uses a mix of fructose and urea, lets do whole-brain clearing in larger animals (like adult rabbits) (11).
- ClearT/T2 and RTF: Formamide-based ClearT/T2 techniques are useful for rapidly clearing small samples, such as mouse embryos, but are less effective for adult brains. Rapid clearing method based on triethanolamine and formamide (RTF) is a variation of ClearT2 that improves clearing performance in adult brain tissues.
While simple immersion methods are pretty easy to carry out – and maintain a tissue’s structural integrity – they don’t remove lipids, which limits the transparency due to light scattering by residual lipids.
Delipidation and Hydration Methods
To get around the limitations of simple immersion, people came up with delipidation methods. These methods improve clearing by removing lipids, the primary source of light scattering. Some examples include (10):
- FACT (fast free-of-acrylamide clearing tissue): An SDS-based clearing method that improves both the speed and quality of tissue clearing, making it suitable for whole-tissue imaging in mouse models. This method has been successful in achieving high-quality 3D imaging.
- OPTIClear: Combines SDS treatment with a novel RI matching solution, making it excellent for clearing human brain tissues without messing up structural integrity.
- SeeDB2: An improvement over the original SeeDB, SeeDB2 uses iohexol and saponin to improve clearing.
- FOCM (ultrafast optical clearing method): Designed for super-fast clearing – you can clear a 300-µm-thick brain slice just two minutes without causing tissue deformation or affecting fluorescent proteins (12).
- Ce3D (clearing-enhanced 3D): Achieves excellent transparency in most mouse organs within 24 hours and offer you a balance between speed and quality.
There’s also the Clear Unobstructed Brain/body Imaging Cocktails (CUBIC) clearing method, which we think deserves its own section.
CUBIC
CUBIC is another hydration/delipidation clearing method that uses urea in combination with aminoalcohols to clear tissues and has become one of the most versatile and widely used aqueous clearing techniques. Although it was originally developed to use a series of amino alcohols to delipidate and decolorize mammalian brains, you don’t have to stick with brains if you don’t want to – you can even use whole-body clearing with light-sheet microscopy if you like (13).
- Mechanism: CUBIC involves a two-step process where the first reagent (CUBIC-R) removes lipids and proteins, and the second reagent (CUBIC-L) clears the remaining structures. This method reduces the RI mismatch within the tissue to make it transparent.
- Application: Works on a variety of tissues, including dense organs like the liver and kidney, as well as brain tissue. Because the method is adaptable, you can use it in a broad range of applications.
- Pros: High transparency achieved and compatible with multiple fluorescent dyes, making multiplex imaging that much easier. It’s also relatively gentle on tissues so there’s less risk of damage compared to solvent-based methods.
- Cons: Slower than solvent-based techniques, especially when you have to work with large or dense tissues – the process can take several days to weeks depending on the sample size and type (7).
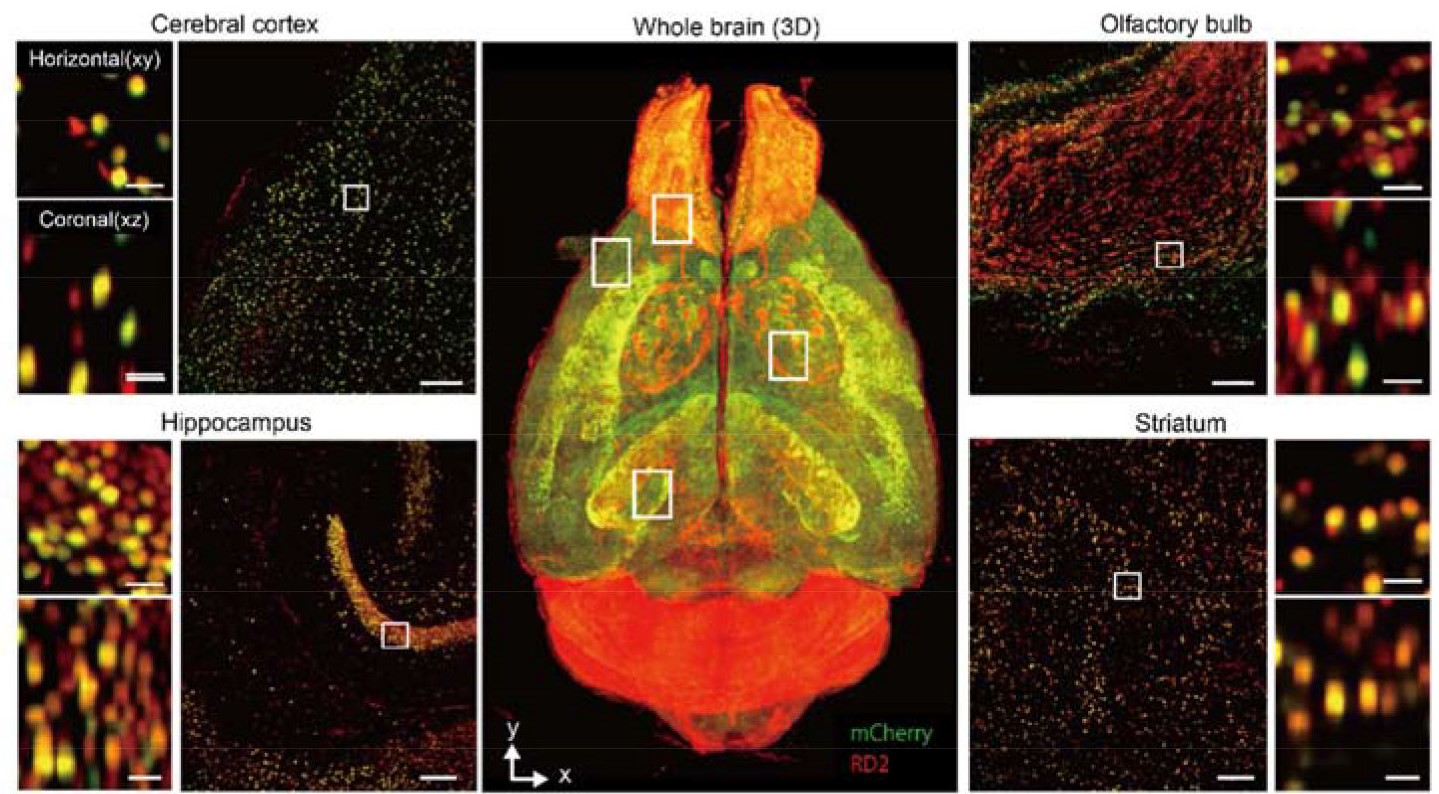
Figure 3. Whole-Brain cleared with CUBIC and Imaged with Light-Sheet Fluorescent Microscopy. Volume-rendered and single-plane images of a brain transduced with AAV-PHP.eB:NSE-H2B-mCherry (mCherry, green) and counterstained by RD2 (red), which is cleared by CUBIC-L/R+. Overlapped signals are shown in yellow. A volume-rendered image is shown in the center. Single-plane and magnified images are shown for cerebral cortex, hippocampus, olfactory bulb, and striatum. Both horizontal (x-y) and coronal (x-z) views are also shown. Scale bars, 200 μm (single-plane image) and 25 μm (magnified image). From Ueda et al. (2020) (7).
Hydrogel-Based Clearing Techniques
Finally, we come to hydrogel-based techniques where you embed your tissue in a supportive hydrogel matrix to preserve those fine structural details you really want to image. These methods open up high-resolution imaging options and excellent tissue preservation, but the processes can be complex and takes a comparatively long time.
CLARITY
Clear Lipid-exchanged Acrylamide-hybridized Rigid Imaging/immunostaining/in situ hybridization-compatible Tissue-Hydrogel (CLARITY) is the clearing method all about preserving tissue structure. It works by embedding the tissue in a hydrogel to stabilize biomolecules while lipids are removed (14). This technique is particularly beloved in neuroscience because it maintains the many, many, fine details of brain tissues, allowing you to look deep inside without losing the details.
- Mechanism: Uses a hydrogel matrix to support the tissue structure. You then remove lipids via electrophoresis, nicely keeping the proteins and nucleic acids in place.
- Applications: Great for brain tissue where preserving the three-dimensional structure of neurons and other cells is critical. It’s also compatible with a wide range of fluorescent dyes and antibodies.
- Pros: Preserves endogenous fluorescence while the hydrogel matrix keeps the tissue intact, making it a good choice for long-term studies.
- Cons: Slow processing time, especially for larger samples. The electrophoretic process can also be tricky to optimize (10).
PACT
Passive CLARITY technique (PACT) is a simplified version of CLARITY that doesn’t rely on electrophoresis to remove lipids – instead, it uses passive diffusion to clear the tissue. One of the goals behind developing PACT was to improve tissue permeability and probe penetration by decreasing gel density (15). PACT was also later combined with PARS (perfusion-assisted agent release in situ) to make whole rodent bodies transparent (which is pretty cool!) (16, 17).
- Mechanism: Uses a hydrogel to embed the tissue, much like CLARITY. However, it relies on passive diffusion to remove lipids so there’s less need for specialized equipment.
- Applications: Particularly useful in neuroscience for imaging brain tissues where maintaining the fine architecture of neural networks is important.
- Pros: Easier and more accessible than CLARITY due to the lack of need for electrophoresis. It still offers good tissue preservation and is compatible with various staining protocols.
- Cons: Slower clearing times due to passive diffusion. The process can take longer for larger or more lipid-rich tissues. Also, weakening the gel architecture to improve permeability means it doesn’t hold tissue integrity as well as other methods (2).
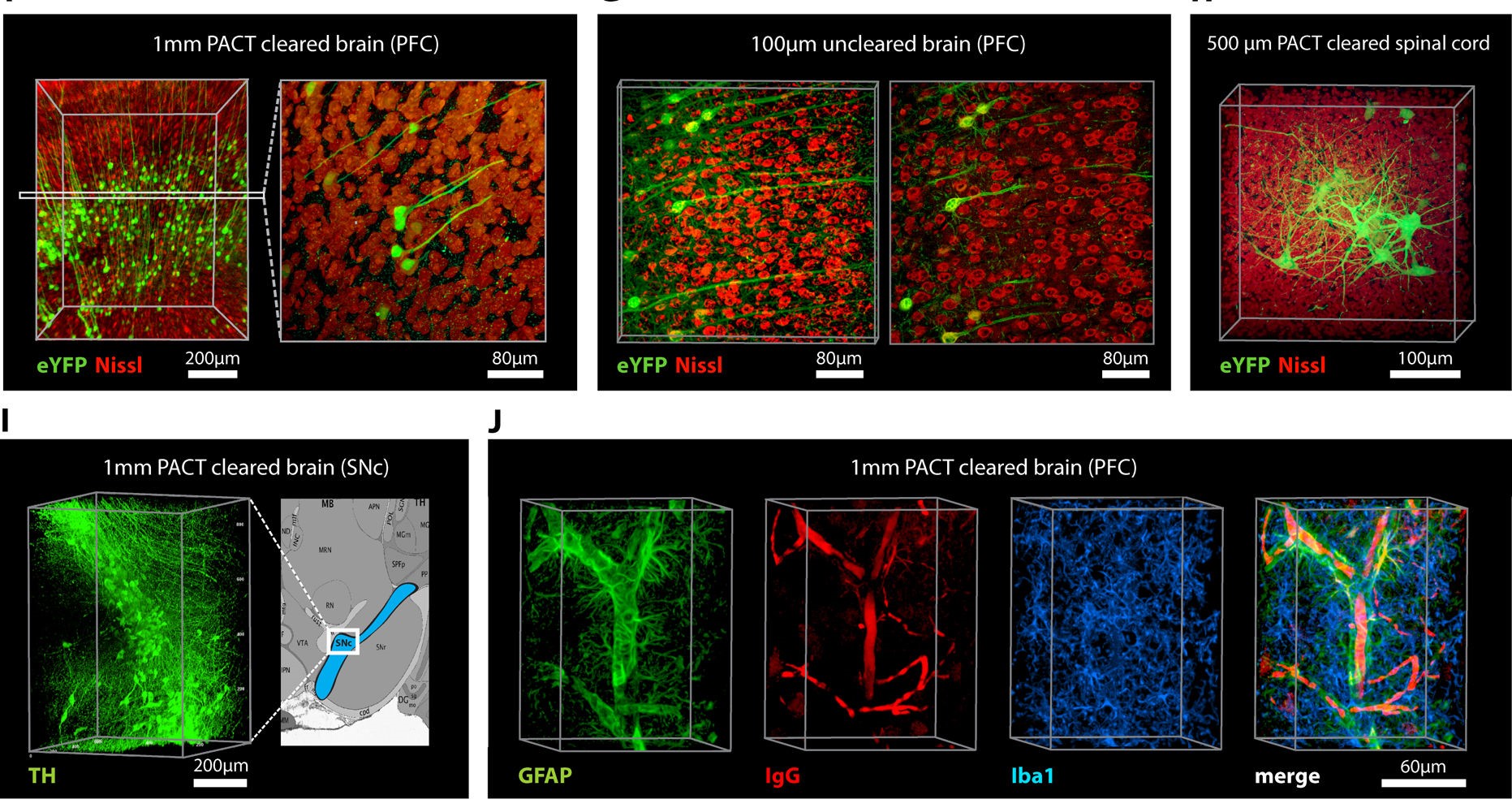
Figure 4. Adult Mouse Sections Cleared with PACT. (F–H) Thy1-eYFP mouse sections stained with Nissl: (F) 1 mm cleared brain slice, prefrontal cortex (PFC) area (left: z = 1 mm imaging stack depth); (G) 100 μm uncleared brain slice, PFC (left: z = 100 μm imaging stack depth); (H) 1 mm spinal cord slice (z = 500 μm). (I) Substantia nigra pars compacta (SNc) of 1 mm mouse brain slice stained with anti-tyrosine hydroxylase (TH) antibody (z = 1 mm). (J) PFC of 1 mm adult mouse brain slices stained with antibodies against GFAP, mouse-IgG, and Iba1 (z = 500 μm) From Yang et al. (2014) (17).
ExM
Expansion Microscopy (ExM) does something different: it physically enlarges the tissue before imaging (18). This expansion allows you to run super-rsolution imaging with standard microscopes, so you can see all of the tiny subcellular structures with incredible detail.
- Mechanism: The tissue is embedded in a swellable polymer. Once the tissue is expanded, the structures inside can be imaged at higher resolutions than would be possible without expansion. This effectively increases the physical distance between molecules.
- Applications: Ideal for high-resolution imaging of subcellular structures such as synapses, protein complexes, and other small features within cells.
- Pros: Provides unmatched resolution and visualization of structures that would otherwise be too small to see. This makes it especially valuable for studying cellular machinery.
- Cons: The process is complex and can result in tissue distortion. Careful calibration is needed to ensure that the expansion is uniform across the sample.
Emerging Clearing Techniques
We will always be on the hunt for better ways to see deeper into tissues and so clearing techniques will inevitably be in a state of constant improvement. New hybrid techniques are being developed that combine the strengths of solvent and aqueous-based methods, for example, which offer faster clearing times, better preservation of fluorescence, and improved compatibility with a multiple of staining protocols.
Unsurprisingly, artificial intelligence (AI) is also being brought into this arena. AI-driven algorithms could optimize clearing protocols by predicting the ideal conditions for different tissue types, which would reduce the trial-and-error aspects inherent to developing these techniques. Additionally, AI could assist in analyzing the massive datasets generated after our 3D imaging of cleared tissues. This would give us more accurate quantification and identification of cellular structures. By combining AI’s “deep 3D histology” (19) with traditional clearing methods, we may all be on the verge of faster, more reliable results from our microscopes. The end goal would be a complete 3D tissue atlas! (20).
Seeing Clearly
Each tissue clearing technique has its strengths and weaknesses. BABB and 3DISCO offer rapid clearing for large samples, CLARITY and CUBIC excel in preserving tissue structure and fluorescence, and PACT and ExM push the boundaries of resolution and tissue preservation.
Which clearing method you choose always depends on your specific needs. Hopefully, this clearing overview helps you to choose the right technique that works best for your setup.
Alomone Lab specializes in developing high-quality molecular detectors for ion channels and other membrane proteins. Consider trying our conjugated antibodies and our conjugated venom-derived toxins in your 3D immunodetection studies. If you don’t find exactly what you are looking for, please refer to our conjugation services. As tissue clearing and 3D immunodetection are complicated extremely time-consuming processes, using a conjugated detector may benefit you with reduced calibration requirements and a significantly shorter protocol.
If you ever need advice on Alomone’s conjugated detectors, you can always drop our scientists a message or have a quick phone call.
References
1. J. A. Dent, A. G. Polson, M. W. Klymkowsky, A whole-mount immunocytochemical analysis of the expression of the intermediate filament protein vimentin in Xenopus. Development 105, 61–74 (1989). DOI: https://doi.org/10.1242/dev.105.1.61.
2. H. R. Ueda, A. Ertürk, K. Chung, V. Gradinaru, A. Chédotal, P. Tomancak, P. J. Keller, Tissue clearing and its applications in neuroscience. Nat Rev Neurosci 21, 61–79 (2020). DOI: https://doi.org/10.1038/s41583-019-0250-1.
3. H.-U. Dodt, U. Leischner, A. Schierloh, N. Jährling, C. P. Mauch, K. Deininger, J. M. Deussing, M. Eder, W. Zieglgänsberger, K. Becker, Ultramicroscopy: three-dimensional visualization of neuronal networks in the whole mouse brain. Nat Methods 4, 331–336 (2007). DOI: https://doi.org/10.1038/nmeth1036.
4. D. S. Foster, A. T. Nguyen, M. Chinta, A. Salhotra, R. E. Jones, S. Mascharak, A. L. Titan, R. C. Ransom, O. L. da Silva, E. Foley, E. Briger, M. T. Longaker, A Clearing Technique to Enhance Endogenous Fluorophores in Skin and Soft Tissue. Sci Rep 9, 15791 (2019). DOI: https://doi.org/10.1038/s41598-019-50359-x.
5. A. Ertürk, C. P. Mauch, F. Hellal, F. Förstner, T. Keck, K. Becker, N. Jährling, H. Steffens, M. Richter, M. Hübener, E. Kramer, F. Kirchhoff, H. U. Dodt, F. Bradke, Three-dimensional imaging of the unsectioned adult spinal cord to assess axon regeneration and glial responses after injury. Nat Med 18, 166–171 (2011). DOI: https://doi.org/10.1038/nm.2600.
6. N. Renier, E. L. Adams, C. Kirst, Z. Wu, R. Azevedo, J. Kohl, A. E. Autry, L. Kadiri, K. Umadevi Venkataraju, Y. Zhou, V. X. Wang, C. Y. Tang, O. Olsen, C. Dulac, P. Osten, M. Tessier-Lavigne, Mapping of Brain Activity by Automated Volume Analysis of Immediate Early Genes. Cell 165, 1789–1802 (2016). DOI: https://doi.org/10.1016/j.cell.2016.05.007.
7. H. R. Ueda, H.-U. Dodt, P. Osten, M. N. Economo, J. Chandrashekar, P. J. Keller, Whole-Brain Profiling of Cells and Circuits in Mammals by Tissue Clearing and Light-Sheet Microscopy. Neuron 106, 369–387 (2020). DOI: https://doi.org/10.1016/j.neuron.2020.03.004.
8. C. Pan, R. Cai, F. P. Quacquarelli, A. Ghasemigharagoz, A. Lourbopoulos, P. Matryba, N. Plesnila, M. Dichgans, F. Hellal, A. Ertürk, Shrinkage-mediated imaging of entire organs and organisms using uDISCO. Nat Methods 13, 859–867 (2016). DOI: https://doi.org/10.1038/nmeth.3964.
9. Q. Li, N. N. Aalling, B. Förstera, A. Ertürk, M. Nedergaard, K. Møllgård, A. L. R. Xavier, Aquaporin 1 and the Na+/K+/2Cl− cotransporter 1 are present in the leptomeningeal vasculature of the adult rodent central nervous system. Fluids Barriers CNS 17, 15 (2020). DOI: https://doi.org/10.1186/s12987-020-0176-z.
10. T. Tian, Z. Yang, X. Li, Tissue clearing technique: Recent progress and biomedical applications. Journal of Anatomy 238, 489–507 (2021). DOI: https://doi.org/10.1111/joa.13309.
11. B. Hou, D. Zhang, S. Zhao, M. Wei, Z. Yang, S. Wang, J. Wang, X. Zhang, B. Liu, L. Fan, Y. Li, Z. Qiu, C. Zhang, T. Jiang, Scalable and DiI-compatible optical clearance of the mammalian brain. Front. Neuroanat. 9 (2015). DOI: https://doi.org/10.3389/fnana.2015.00019.
12. Q.-H. Shan, X.-Y. Qin, N. Zhou, C. Huang, Y. Wang, P. Chen, J.-N. Zhou, A method for ultrafast tissue clearing that preserves fluorescence for multimodal and longitudinal brain imaging. BMC Biology 20, 77 (2022). DOI: https://doi.org/10.1186/s12915-022-01275-6.
13. E. A. Susaki, K. Tainaka, D. Perrin, H. Yukinaga, A. Kuno, H. R. Ueda, Advanced CUBIC protocols for whole-brain and whole-body clearing and imaging. Nat Protoc 10, 1709–1727 (2015). DOI: https://doi.org/10.1038/nprot.2015.085.
14. K. Chung, J. Wallace, S.-Y. Kim, S. Kalyanasundaram, A. S. Andalman, T. J. Davidson, J. J. Mirzabekov, K. A. Zalocusky, J. Mattis, A. K. Denisin, S. Pak, H. Bernstein, C. Ramakrishnan, L. Grosenick, V. Gradinaru, K. Deisseroth, Structural and molecular interrogation of intact biological systems. Nature 497, 332–337 (2013). DOI: https://doi.org/10.1038/nature12107.
15. R. Tomer, L. Ye, B. Hsueh, K. Deisseroth, Advanced CLARITY for rapid and high-resolution imaging of intact tissues. Nat Protoc 9, 1682–1697 (2014). DOI: https://doi.org/10.1038/nprot.2014.123.
16. J. B. Treweek, K. Y. Chan, N. C. Flytzanis, B. Yang, B. E. Deverman, A. Greenbaum, A. Lignell, C. Xiao, L. Cai, M. S. Ladinsky, P. J. Bjorkman, C. C. Fowlkes, V. Gradinaru, Whole-body tissue stabilization and selective extractions via tissue-hydrogel hybrids for high-resolution intact circuit mapping and phenotyping. Nat Protoc 10, 1860–1896 (2015). DOI: https://doi.org/10.1038/nprot.2015.122.
17. B. Yang, J. B. Treweek, R. P. Kulkarni, B. E. Deverman, C.-K. Chen, E. Lubeck, S. Shah, L. Cai, V. Gradinaru, Single-Cell Phenotyping within Transparent Intact Tissue through Whole-Body Clearing. Cell 158, 945–958 (2014). DOI: https://doi.org/10.1016/j.cell.2014.07.017.
18. T. C. Murakami, T. Mano, S. Saikawa, S. A. Horiguchi, D. Shigeta, K. Baba, H. Sekiya, Y. Shimizu, K. F. Tanaka, H. Kiyonari, M. Iino, H. Mochizuki, K. Tainaka, H. R. Ueda, A three-dimensional single-cell-resolution whole-brain atlas using CUBIC-X expansion microscopy and tissue clearing. Nat Neurosci 21, 625–637 (2018). DOI: https://doi.org/10.1038/s41593-018-0109-1.
19. A. Ertürk, Deep 3D histology powered by tissue clearing, omics and AI. Nat Methods 21, 1153–1165 (2024). DOI: https://doi.org/10.1038/s41592-024-02327-1.20. R. Diogo, N. Siomava, Y. Gitton, Development of human limb muscles based on whole-mount immunostaining and the links between ontogeny and evolution. Development 146, dev180349 (2019). DOI: https://doi.org/10.1242/dev.180349.